ULN2803A (Rev. F) - Texas Instruments - Farnell Element 14
- Revenir à l'accueil

Farnell Element 14 :
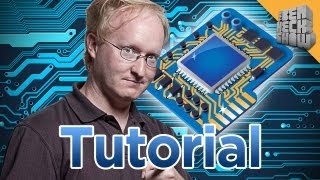
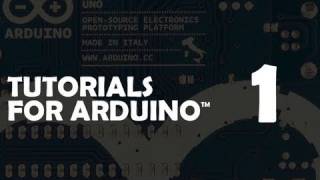
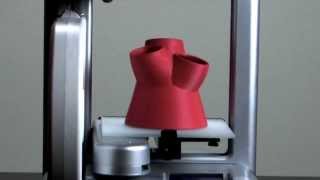
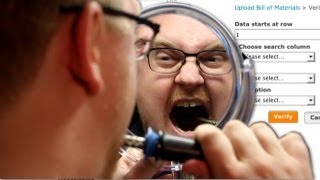
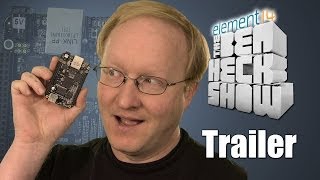
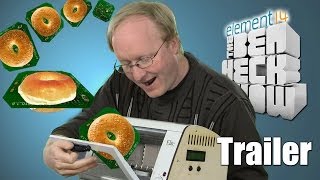
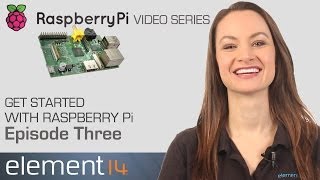
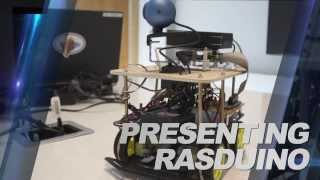
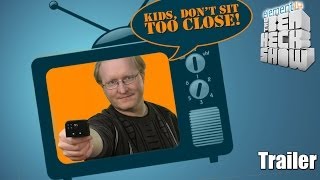
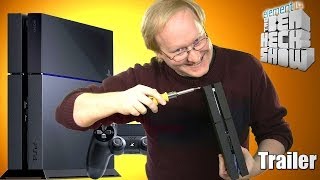
See the trailer for the next exciting episode of The Ben Heck show. Check back on Friday to be among the first to see the exclusive full show on element…
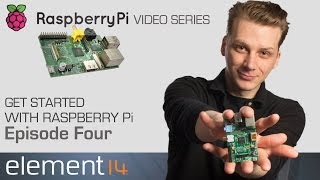
Connect your Raspberry Pi to a breadboard, download some code and create a push-button audio play project.
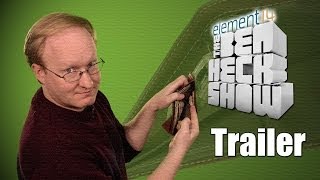
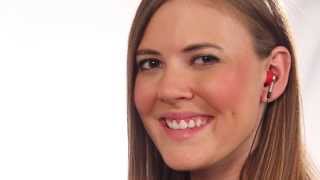
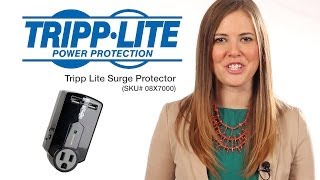
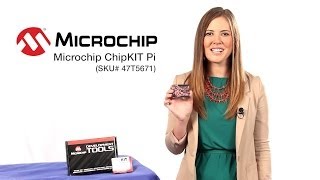
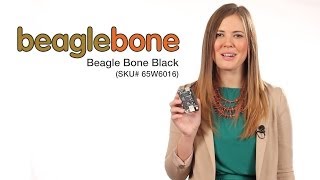
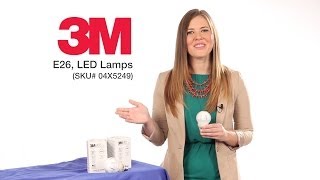
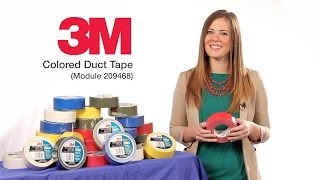
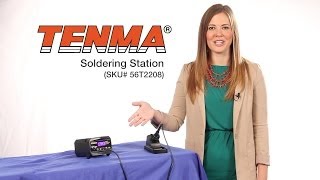
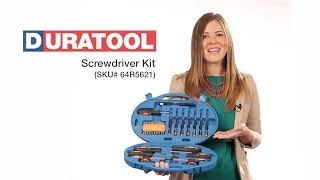
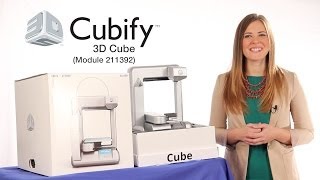
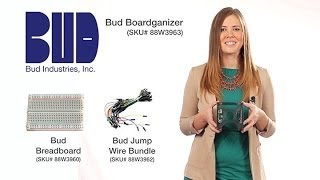
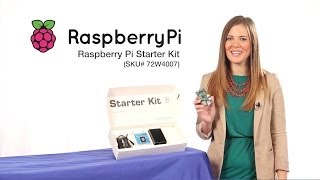
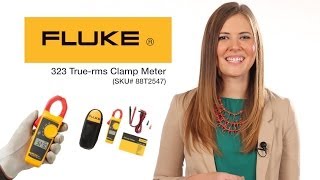
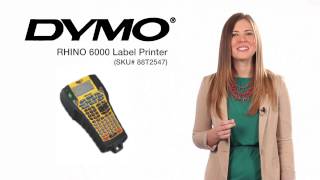
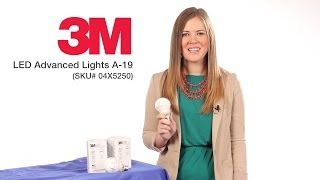
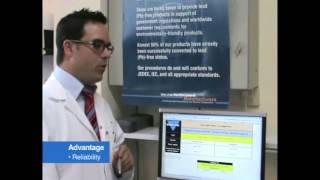
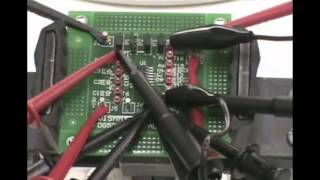
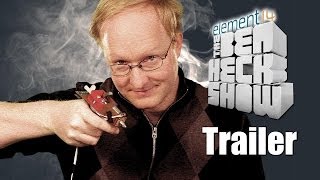
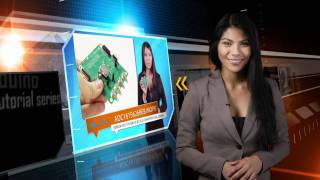
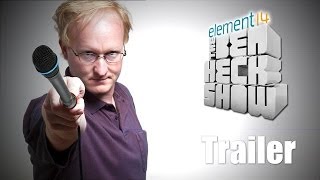
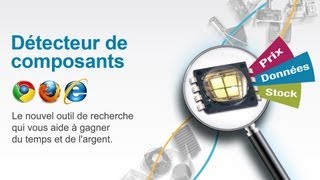
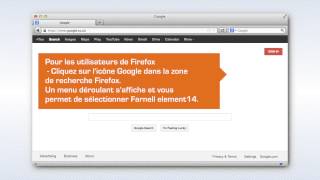
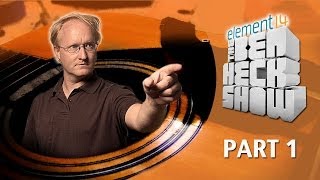
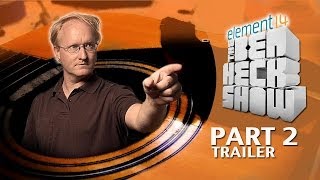
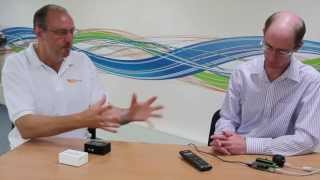
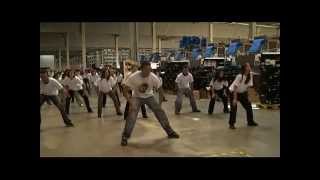
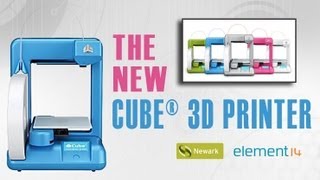










Puce électronique / Microchip :
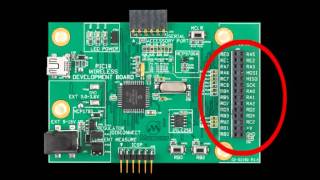
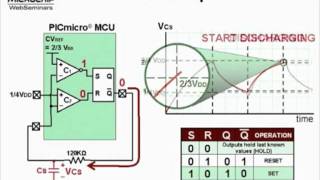
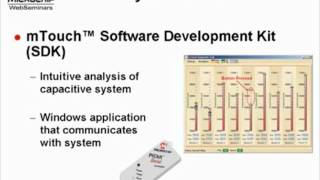
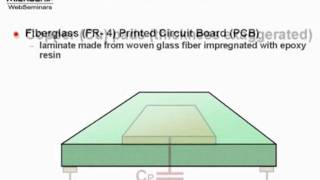
Sans fil - Wireless :
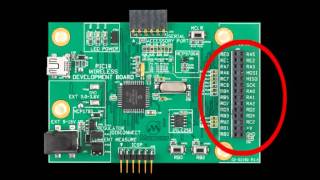
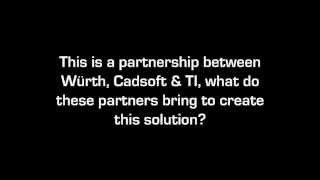
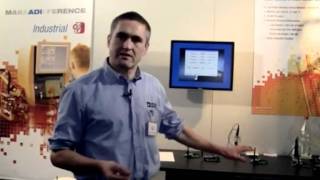
Texas instrument :
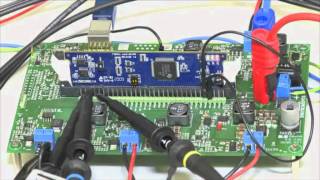
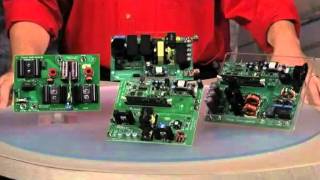
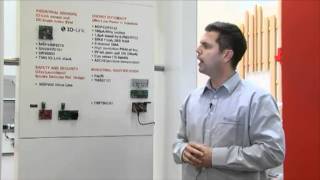
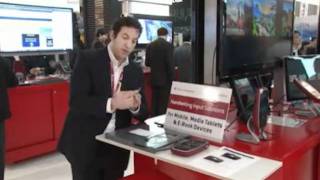
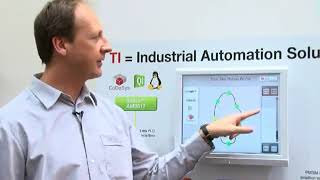
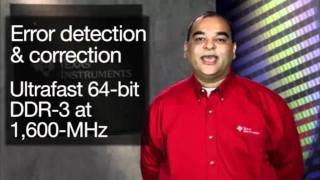
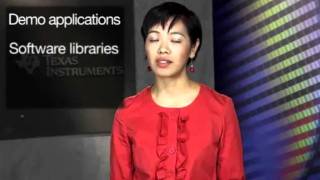
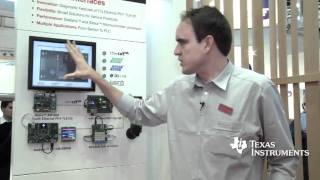
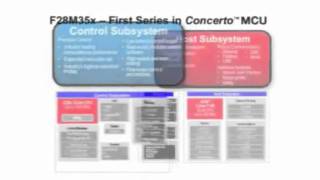
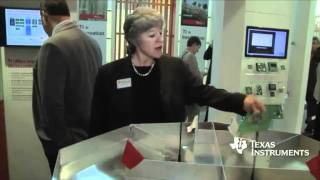
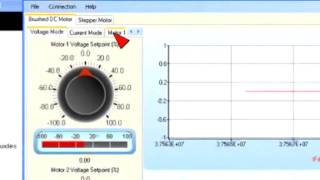
Ordinateurs :
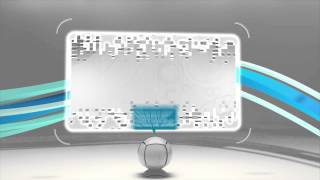
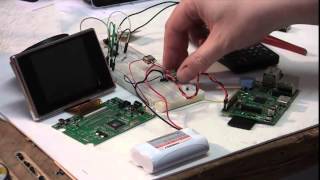
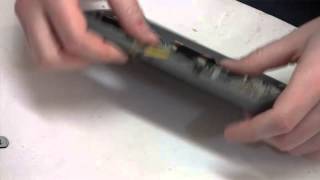
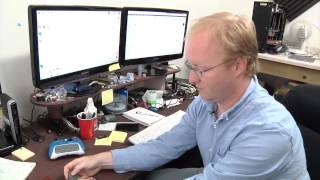
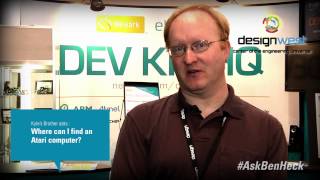
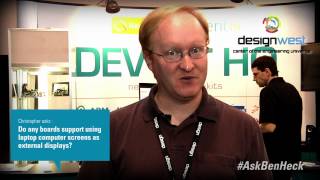
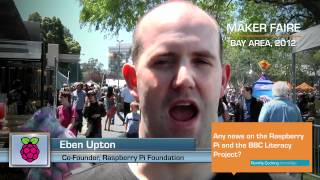
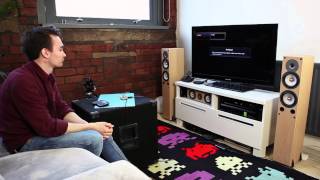
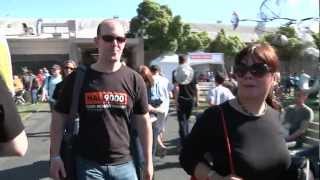
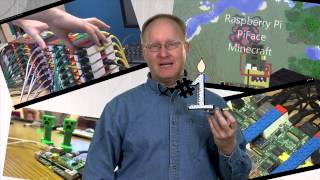
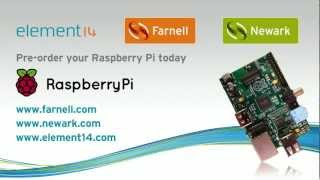
Logiciels :
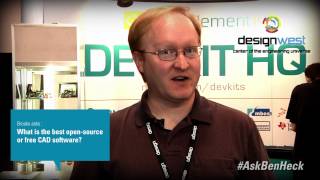
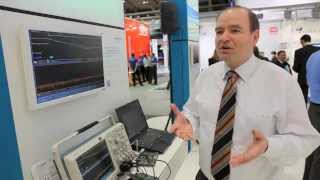
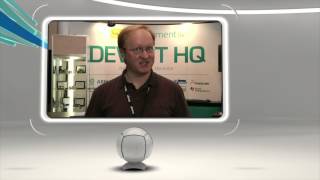
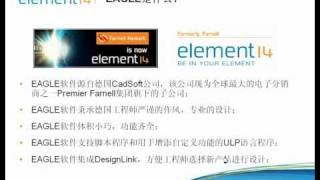
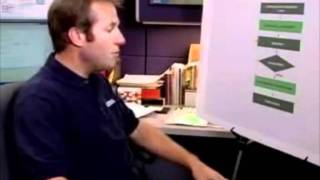
Tutoriels :
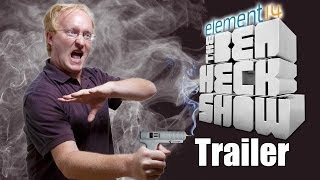
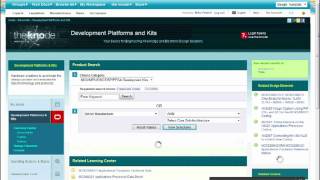
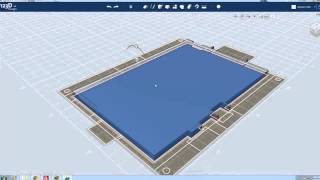
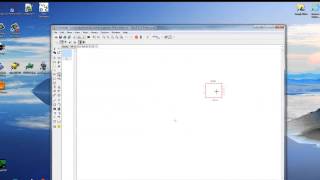
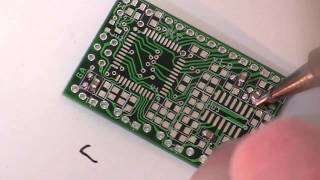
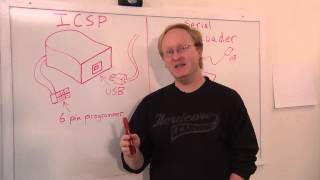
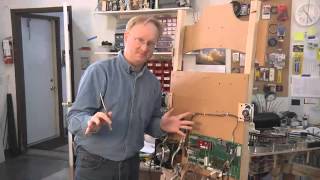
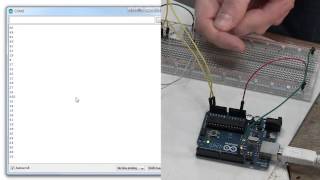
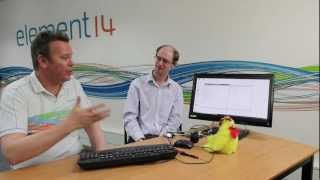
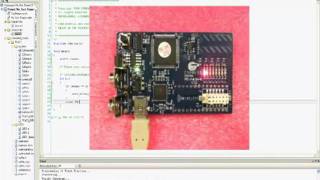
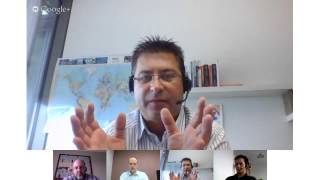
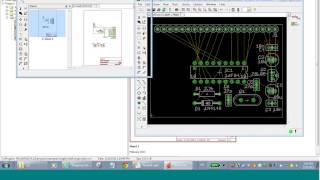
Autres documentations :
Analog-Devices-Convo..> 09-Sep-2014 08:26 2.1M
Analog-Devices-Convo..> 09-Sep-2014 08:25 2.2M
Analog-Devices-Convo..> 09-Sep-2014 08:25 2.2M
Analog-Devices-ADMC4..> 09-Sep-2014 08:23 2.3M
Analog-Devices-Wi-Fi..> 09-Sep-2014 08:23 2.3M
Analog-Devices-ADMC2..> 09-Sep-2014 08:21 2.4M
Analog-Devices-ADC-S..> 09-Sep-2014 08:21 2.4M
Analog-Devices-Visua..> 09-Sep-2014 08:18 2.5M
Analog-Devices-ANF32..> 09-Sep-2014 08:18 2.6M
Farnell-Compensating..> 09-Sep-2014 08:16 2.6M
Farnell-Compensating..> 09-Sep-2014 08:16 2.6M
Farnell-LM7805-Fairc..> 09-Sep-2014 08:13 2.7M
Farnell-AD620-Rev-H-..> 09-Sep-2014 08:13 2.6M
Farnell-Datasheet-FT..> 09-Sep-2014 08:10 2.8M
Farnell-MAX4661-MAX4..> 09-Sep-2014 08:10 2.8M
Farnell-OPA627-Texas..> 09-Sep-2014 08:08 2.8M
Farnell-REF19x-Serie..> 09-Sep-2014 08:08 2.8M
Farnell-Data-Sheet-M..> 09-Sep-2014 08:05 2.8M
Farnell-ULN2803A-Rev..> 09-Sep-2014 08:05 2.9M
Analog-Devices-Digit..> 08-Sep-2014 18:03 2.0M
Analog-Devices-Digit..> 08-Sep-2014 18:02 2.1M
Analog-Devices-Basic..> 08-Sep-2014 17:49 1.9M
Farnell-AD9833-Rev-E..> 08-Sep-2014 17:49 1.8M
Farnell-The-Discrete..> 08-Sep-2014 17:44 1.8M
Electronique-Basic-o..> 08-Sep-2014 17:43 1.8M
Analog-Devices-AN300..> 08-Sep-2014 17:42 2.0M
Analog-Devices-The-C..> 08-Sep-2014 17:41 1.9M
Analog-Devices-Intro..> 08-Sep-2014 17:39 1.9M
Analog-Devices-Compl..> 08-Sep-2014 17:38 2.0M
Analog-Devices-Gloss..> 08-Sep-2014 17:36 2.0M
Farnell-ADuM1300-ADu..> 08-Sep-2014 08:11 1.7M
Farnell-AD586BRZ-Ana..> 08-Sep-2014 08:09 1.6M
Farnell-ADuM1200-ADu..> 08-Sep-2014 08:09 1.6M
Farnell-NA555-NE555-..> 08-Sep-2014 07:51 1.5M
Farnell-AD9834-Rev-D..> 08-Sep-2014 07:32 1.2M
Farnell-MSP430F15x-M..> 08-Sep-2014 07:32 1.3M
Farnell-AD736-Rev-I-..> 08-Sep-2014 07:31 1.3M
Farnell-AD8307-Data-..> 08-Sep-2014 07:30 1.3M
Farnell-Single-Chip-..> 08-Sep-2014 07:30 1.5M
Farnell-Quadruple-2-..> 08-Sep-2014 07:29 1.5M
Farnell-ADE7758-Rev-..> 08-Sep-2014 07:28 1.7M
Farnell-MAX3221-Rev-..> 08-Sep-2014 07:28 1.8M
Farnell-USB-to-Seria..> 08-Sep-2014 07:27 2.0M
Farnell-AD8313-Analo..> 08-Sep-2014 07:26 2.0M
Farnell-SN54HC164-SN..> 08-Sep-2014 07:25 2.0M
Farnell-AD8310-Analo..> 08-Sep-2014 07:24 2.1M
Farnell-AD8361-Rev-D..> 08-Sep-2014 07:23 2.1M
Farnell-2N3906-Fairc..> 08-Sep-2014 07:22 2.1M
Farnell-AD584-Rev-C-..> 08-Sep-2014 07:20 2.2M
Farnell-ADE7753-Rev-..> 08-Sep-2014 07:20 2.3M
Farnell-TLV320AIC23B..> 08-Sep-2014 07:18 2.4M
![[TXT]](http://www.audentia-gestion.fr/icons/text.gif)
Farnell-NA555-NE555-..> 08-Sep-2014 07:33 1.5M
![[TXT]](http://www.audentia-gestion.fr/icons/text.gif)
Farnell-AD9834-Rev-D..> 08-Sep-2014 07:32 1.2M
![[TXT]](http://www.audentia-gestion.fr/icons/text.gif)
Farnell-MSP430F15x-M..> 08-Sep-2014 07:32 1.3M
![[TXT]](http://www.audentia-gestion.fr/icons/text.gif)
Farnell-AD736-Rev-I-..> 08-Sep-2014 07:31 1.3M
![[TXT]](http://www.audentia-gestion.fr/icons/text.gif)
Farnell-AD8307-Data-..> 08-Sep-2014 07:30 1.3M
![[TXT]](http://www.audentia-gestion.fr/icons/text.gif)
Farnell-Single-Chip-..> 08-Sep-2014 07:30 1.5M
![[TXT]](http://www.audentia-gestion.fr/icons/text.gif)
Farnell-Quadruple-2-..> 08-Sep-2014 07:29 1.5M
![[TXT]](http://www.audentia-gestion.fr/icons/text.gif)
Farnell-ADE7758-Rev-..> 08-Sep-2014 07:28 1.7M
![[TXT]](http://www.audentia-gestion.fr/icons/text.gif)
Farnell-MAX3221-Rev-..> 08-Sep-2014 07:28 1.8M
![[TXT]](http://www.audentia-gestion.fr/icons/text.gif)
Farnell-USB-to-Seria..> 08-Sep-2014 07:27 2.0M
![[TXT]](http://www.audentia-gestion.fr/icons/text.gif)
Farnell-AD8313-Analo..> 08-Sep-2014 07:26 2.0M
![[TXT]](http://www.audentia-gestion.fr/icons/text.gif)
Farnell-SN54HC164-SN..> 08-Sep-2014 07:25 2.0M
![[TXT]](http://www.audentia-gestion.fr/icons/text.gif)
Farnell-AD8310-Analo..> 08-Sep-2014 07:24 2.1M
![[TXT]](http://www.audentia-gestion.fr/icons/text.gif)
Farnell-AD8361-Rev-D..> 08-Sep-2014 07:23 2.1M
![[TXT]](http://www.audentia-gestion.fr/icons/text.gif)
Farnell-2N3906-Fairc..> 08-Sep-2014 07:22 2.1M
![[TXT]](http://www.audentia-gestion.fr/icons/text.gif)
Farnell-AD584-Rev-C-..> 08-Sep-2014 07:20 2.2M
![[TXT]](http://www.audentia-gestion.fr/icons/text.gif)
Farnell-ADE7753-Rev-..> 08-Sep-2014 07:20 2.3M
![[TXT]](http://www.audentia-gestion.fr/icons/text.gif)
Farnell-TLV320AIC23B..> 08-Sep-2014 07:18 2.4M
![[TXT]](http://www.audentia-gestion.fr/icons/text.gif)
Farnell-AD586BRZ-Ana..> 08-Sep-2014 07:17 1.6M
![[TXT]](http://www.audentia-gestion.fr/icons/text.gif)
Farnell-STM32F405xxS..> 27-Aug-2014 18:27 1.8M
Farnell-MSP430-Hardw..> 29-Jul-2014 10:36 1.1M
![[TXT]](http://www.audentia-gestion.fr/icons/text.gif)
Farnell-LM324-Texas-..> 29-Jul-2014 10:32 1.5M
![[TXT]](http://www.audentia-gestion.fr/icons/text.gif)
Farnell-LM386-Low-Vo..> 29-Jul-2014 10:32 1.5M
![[TXT]](http://www.audentia-gestion.fr/icons/text.gif)
Farnell-NE5532-Texas..> 29-Jul-2014 10:32 1.5M
![[TXT]](http://www.audentia-gestion.fr/icons/text.gif)
Farnell-Hex-Inverter..> 29-Jul-2014 10:31 875K
![[TXT]](http://www.audentia-gestion.fr/icons/text.gif)
Farnell-AT90USBKey-H..> 29-Jul-2014 10:31 902K
![[TXT]](http://www.audentia-gestion.fr/icons/text.gif)
Farnell-AT89C5131-Ha..> 29-Jul-2014 10:31 1.2M
![[TXT]](http://www.audentia-gestion.fr/icons/text.gif)
Farnell-MSP-EXP430F5..> 29-Jul-2014 10:31 1.2M
![[TXT]](http://www.audentia-gestion.fr/icons/text.gif)
Farnell-Explorer-16-..> 29-Jul-2014 10:31 1.3M
![[TXT]](http://www.audentia-gestion.fr/icons/text.gif)
Farnell-TMP006EVM-Us..> 29-Jul-2014 10:30 1.3M
![[TXT]](http://www.audentia-gestion.fr/icons/text.gif)
Farnell-Gertboard-Us..> 29-Jul-2014 10:30 1.4M
![[TXT]](http://www.audentia-gestion.fr/icons/text.gif)
Farnell-LMP91051-Use..> 29-Jul-2014 10:30 1.4M
![[TXT]](http://www.audentia-gestion.fr/icons/text.gif)
Farnell-Thermometre-..> 29-Jul-2014 10:30 1.4M
![[TXT]](http://www.audentia-gestion.fr/icons/text.gif)
Farnell-user-manuel-..> 29-Jul-2014 10:29 1.5M
![[TXT]](http://www.audentia-gestion.fr/icons/text.gif)
Farnell-fx-3650P-fx-..> 29-Jul-2014 10:29 1.5M
![[TXT]](http://www.audentia-gestion.fr/icons/text.gif)
Farnell-2-GBPS-Diffe..> 28-Jul-2014 17:42 2.7M
![[TXT]](http://www.audentia-gestion.fr/icons/text.gif)
Farnell-LMT88-2.4V-1..> 28-Jul-2014 17:42 2.8M
![[TXT]](http://www.audentia-gestion.fr/icons/text.gif)
Farnell-Octal-Genera..> 28-Jul-2014 17:42 2.8M
![[TXT]](http://www.audentia-gestion.fr/icons/text.gif)
Farnell-Dual-MOSFET-..> 28-Jul-2014 17:41 2.8M
![[TXT]](http://www.audentia-gestion.fr/icons/text.gif)
Farnell-TLV320AIC325..> 28-Jul-2014 17:41 2.9M
![[TXT]](http://www.audentia-gestion.fr/icons/text.gif)
Farnell-SN54LV4053A-..> 28-Jul-2014 17:20 5.9M
![[TXT]](http://www.audentia-gestion.fr/icons/text.gif)
Farnell-TAS1020B-USB..> 28-Jul-2014 17:19 6.2M
![[TXT]](http://www.audentia-gestion.fr/icons/text.gif)
Farnell-TPS40060-Wid..> 28-Jul-2014 17:19 6.3M
![[TXT]](http://www.audentia-gestion.fr/icons/text.gif)
Farnell-TL082-Wide-B..> 28-Jul-2014 17:16 6.3M
![[TXT]](http://www.audentia-gestion.fr/icons/text.gif)
Farnell-RF-short-tra..> 28-Jul-2014 17:16 6.3M
![[TXT]](http://www.audentia-gestion.fr/icons/text.gif)
Farnell-maxim-integr..> 28-Jul-2014 17:14 6.4M
![[TXT]](http://www.audentia-gestion.fr/icons/text.gif)
Farnell-TSV6390-TSV6..> 28-Jul-2014 17:14 6.4M
![[TXT]](http://www.audentia-gestion.fr/icons/text.gif)
Farnell-Fast-Charge-..> 28-Jul-2014 17:12 6.4M
![[TXT]](http://www.audentia-gestion.fr/icons/text.gif)
Farnell-NVE-datashee..> 28-Jul-2014 17:12 6.5M
![[TXT]](http://www.audentia-gestion.fr/icons/text.gif)
Farnell-Excalibur-Hi..> 28-Jul-2014 17:10 2.4M
![[TXT]](http://www.audentia-gestion.fr/icons/text.gif)
Farnell-Excalibur-Hi..> 28-Jul-2014 17:10 2.4M
![[TXT]](http://www.audentia-gestion.fr/icons/text.gif)
Farnell-REF102-10V-P..> 28-Jul-2014 17:09 2.4M
![[TXT]](http://www.audentia-gestion.fr/icons/text.gif)
Farnell-TMS320F28055..> 28-Jul-2014 17:09 2.7M
![[TXT]](http://www.audentia-gestion.fr/icons/text.gif)
Farnell-MULTICOMP-Ra..> 22-Jul-2014 12:35 5.9M
![[TXT]](http://www.audentia-gestion.fr/icons/text.gif)
Farnell-RASPBERRY-PI..> 22-Jul-2014 12:35 5.9M
![[TXT]](http://www.audentia-gestion.fr/icons/text.gif)
Farnell-Dremel-Exper..> 22-Jul-2014 12:34 1.6M
![[TXT]](http://www.audentia-gestion.fr/icons/text.gif)
Farnell-STM32F103x8-..> 22-Jul-2014 12:33 1.6M
![[TXT]](http://www.audentia-gestion.fr/icons/text.gif)
Farnell-BD6xxx-PDF.htm 22-Jul-2014 12:33 1.6M
![[TXT]](http://www.audentia-gestion.fr/icons/text.gif)
Farnell-L78S-STMicro..> 22-Jul-2014 12:32 1.6M
![[TXT]](http://www.audentia-gestion.fr/icons/text.gif)
Farnell-RaspiCam-Doc..> 22-Jul-2014 12:32 1.6M
![[TXT]](http://www.audentia-gestion.fr/icons/text.gif)
Farnell-SB520-SB5100..> 22-Jul-2014 12:32 1.6M
![[TXT]](http://www.audentia-gestion.fr/icons/text.gif)
Farnell-iServer-Micr..> 22-Jul-2014 12:32 1.6M
![[TXT]](http://www.audentia-gestion.fr/icons/text.gif)
Farnell-LUMINARY-MIC..> 22-Jul-2014 12:31 3.6M
![[TXT]](http://www.audentia-gestion.fr/icons/text.gif)
Farnell-TEXAS-INSTRU..> 22-Jul-2014 12:31 2.4M
![[TXT]](http://www.audentia-gestion.fr/icons/text.gif)
Farnell-TEXAS-INSTRU..> 22-Jul-2014 12:30 4.6M
![[TXT]](http://www.audentia-gestion.fr/icons/text.gif)
Farnell-CLASS 1-or-2..> 22-Jul-2014 12:30 4.7M
![[TXT]](http://www.audentia-gestion.fr/icons/text.gif)
Farnell-TEXAS-INSTRU..> 22-Jul-2014 12:29 4.8M
![[TXT]](http://www.audentia-gestion.fr/icons/text.gif)
Farnell-Evaluating-t..> 22-Jul-2014 12:28 4.9M
![[TXT]](http://www.audentia-gestion.fr/icons/text.gif)
Farnell-LM3S6952-Mic..> 22-Jul-2014 12:27 5.9M
![[TXT]](http://www.audentia-gestion.fr/icons/text.gif)
Farnell-Keyboard-Mou..> 22-Jul-2014 12:27 5.9M
Farnell-Full-Datashe..> 15-Jul-2014 17:08 951K
![[TXT]](http://www.audentia-gestion.fr/icons/text.gif)
Farnell-pmbta13_pmbt..> 15-Jul-2014 17:06 959K
![[TXT]](http://www.audentia-gestion.fr/icons/text.gif)
Farnell-EE-SPX303N-4..> 15-Jul-2014 17:06 969K
![[TXT]](http://www.audentia-gestion.fr/icons/text.gif)
Farnell-Datasheet-NX..> 15-Jul-2014 17:06 1.0M
![[TXT]](http://www.audentia-gestion.fr/icons/text.gif)
Farnell-Datasheet-Fa..> 15-Jul-2014 17:05 1.0M
![[TXT]](http://www.audentia-gestion.fr/icons/text.gif)
Farnell-MIDAS-un-tra..> 15-Jul-2014 17:05 1.0M
![[TXT]](http://www.audentia-gestion.fr/icons/text.gif)
Farnell-SERIAL-TFT-M..> 15-Jul-2014 17:05 1.0M
![[TXT]](http://www.audentia-gestion.fr/icons/text.gif)
Farnell-MCOC1-Farnel..> 15-Jul-2014 17:05 1.0M
![[TXT]](http://www.audentia-gestion.fr/icons/text.gif)
Farnell-TMR-2-series..> 15-Jul-2014 16:48 787K
![[TXT]](http://www.audentia-gestion.fr/icons/text.gif)
Farnell-DC-DC-Conver..> 15-Jul-2014 16:48 781K
![[TXT]](http://www.audentia-gestion.fr/icons/text.gif)
Farnell-Full-Datashe..> 15-Jul-2014 16:47 803K
![[TXT]](http://www.audentia-gestion.fr/icons/text.gif)
Farnell-TMLM-Series-..> 15-Jul-2014 16:47 810K
![[TXT]](http://www.audentia-gestion.fr/icons/text.gif)
Farnell-TEL-5-Series..> 15-Jul-2014 16:47 814K
![[TXT]](http://www.audentia-gestion.fr/icons/text.gif)
Farnell-TXL-series-t..> 15-Jul-2014 16:47 829K
![[TXT]](http://www.audentia-gestion.fr/icons/text.gif)
Farnell-TEP-150WI-Se..> 15-Jul-2014 16:47 837K
![[TXT]](http://www.audentia-gestion.fr/icons/text.gif)
Farnell-AC-DC-Power-..> 15-Jul-2014 16:47 845K
![[TXT]](http://www.audentia-gestion.fr/icons/text.gif)
Farnell-TIS-Instruct..> 15-Jul-2014 16:47 845K
![[TXT]](http://www.audentia-gestion.fr/icons/text.gif)
Farnell-TOS-tracopow..> 15-Jul-2014 16:47 852K
![[TXT]](http://www.audentia-gestion.fr/icons/text.gif)
Farnell-TCL-DC-traco..> 15-Jul-2014 16:46 858K
![[TXT]](http://www.audentia-gestion.fr/icons/text.gif)
Farnell-TIS-series-t..> 15-Jul-2014 16:46 875K
![[TXT]](http://www.audentia-gestion.fr/icons/text.gif)
Farnell-TMR-2-Series..> 15-Jul-2014 16:46 897K
![[TXT]](http://www.audentia-gestion.fr/icons/text.gif)
Farnell-TMR-3-WI-Ser..> 15-Jul-2014 16:46 939K
![[TXT]](http://www.audentia-gestion.fr/icons/text.gif)
Farnell-TEN-8-WI-Ser..> 15-Jul-2014 16:46 939K
![[TXT]](http://www.audentia-gestion.fr/icons/text.gif)
Farnell-Full-Datashe..> 15-Jul-2014 16:46 947K
Farnell-HIP4081A-Int..> 07-Jul-2014 19:47 1.0M
![[TXT]](http://www.audentia-gestion.fr/icons/text.gif)
Farnell-ISL6251-ISL6..> 07-Jul-2014 19:47 1.1M
![[TXT]](http://www.audentia-gestion.fr/icons/text.gif)
Farnell-DG411-DG412-..> 07-Jul-2014 19:47 1.0M
![[TXT]](http://www.audentia-gestion.fr/icons/text.gif)
Farnell-3367-ARALDIT..> 07-Jul-2014 19:46 1.2M
![[TXT]](http://www.audentia-gestion.fr/icons/text.gif)
Farnell-ICM7228-Inte..> 07-Jul-2014 19:46 1.1M
![[TXT]](http://www.audentia-gestion.fr/icons/text.gif)
Farnell-Data-Sheet-K..> 07-Jul-2014 19:46 1.2M
![[TXT]](http://www.audentia-gestion.fr/icons/text.gif)
Farnell-Silica-Gel-M..> 07-Jul-2014 19:46 1.2M
![[TXT]](http://www.audentia-gestion.fr/icons/text.gif)
Farnell-TKC2-Dusters..> 07-Jul-2014 19:46 1.2M
![[TXT]](http://www.audentia-gestion.fr/icons/text.gif)
Farnell-CRC-HANDCLEA..> 07-Jul-2014 19:46 1.2M
![[TXT]](http://www.audentia-gestion.fr/icons/text.gif)
Farnell-760G-French-..> 07-Jul-2014 19:45 1.2M
![[TXT]](http://www.audentia-gestion.fr/icons/text.gif)
Farnell-Decapant-KF-..> 07-Jul-2014 19:45 1.2M
![[TXT]](http://www.audentia-gestion.fr/icons/text.gif)
Farnell-1734-ARALDIT..> 07-Jul-2014 19:45 1.2M
![[TXT]](http://www.audentia-gestion.fr/icons/text.gif)
Farnell-Araldite-Fus..> 07-Jul-2014 19:45 1.2M
![[TXT]](http://www.audentia-gestion.fr/icons/text.gif)
Farnell-fiche-de-don..> 07-Jul-2014 19:44 1.4M
![[TXT]](http://www.audentia-gestion.fr/icons/text.gif)
Farnell-safety-data-..> 07-Jul-2014 19:44 1.4M
![[TXT]](http://www.audentia-gestion.fr/icons/text.gif)
Farnell-A-4-Hardener..> 07-Jul-2014 19:44 1.4M
![[TXT]](http://www.audentia-gestion.fr/icons/text.gif)
Farnell-CC-Debugger-..> 07-Jul-2014 19:44 1.5M
![[TXT]](http://www.audentia-gestion.fr/icons/text.gif)
Farnell-MSP430-Hardw..> 07-Jul-2014 19:43 1.8M
![[TXT]](http://www.audentia-gestion.fr/icons/text.gif)
Farnell-SmartRF06-Ev..> 07-Jul-2014 19:43 1.6M
![[TXT]](http://www.audentia-gestion.fr/icons/text.gif)
Farnell-CC2531-USB-H..> 07-Jul-2014 19:43 1.8M
![[TXT]](http://www.audentia-gestion.fr/icons/text.gif)
Farnell-Alimentation..> 07-Jul-2014 19:43 1.8M
![[TXT]](http://www.audentia-gestion.fr/icons/text.gif)
Farnell-BK889B-PONT-..> 07-Jul-2014 19:42 1.8M
![[TXT]](http://www.audentia-gestion.fr/icons/text.gif)
Farnell-User-Guide-M..> 07-Jul-2014 19:41 2.0M
![[TXT]](http://www.audentia-gestion.fr/icons/text.gif)
Farnell-T672-3000-Se..> 07-Jul-2014 19:41 2.0M
Farnell-0050375063-D..> 18-Jul-2014 17:03 2.5M
![[TXT]](http://www.audentia-gestion.fr/icons/text.gif)
Farnell-Mini-Fit-Jr-..> 18-Jul-2014 17:03 2.5M
![[TXT]](http://www.audentia-gestion.fr/icons/text.gif)
Farnell-43031-0002-M..> 18-Jul-2014 17:03 2.5M
![[TXT]](http://www.audentia-gestion.fr/icons/text.gif)
Farnell-0433751001-D..> 18-Jul-2014 17:02 2.5M
![[TXT]](http://www.audentia-gestion.fr/icons/text.gif)
Farnell-Cube-3D-Prin..> 18-Jul-2014 17:02 2.5M
![[TXT]](http://www.audentia-gestion.fr/icons/text.gif)
Farnell-MTX-Compact-..> 18-Jul-2014 17:01 2.5M
![[TXT]](http://www.audentia-gestion.fr/icons/text.gif)
Farnell-MTX-3250-MTX..> 18-Jul-2014 17:01 2.5M
![[TXT]](http://www.audentia-gestion.fr/icons/text.gif)
Farnell-ATtiny26-L-A..> 18-Jul-2014 17:00 2.6M
![[TXT]](http://www.audentia-gestion.fr/icons/text.gif)
Farnell-MCP3421-Micr..> 18-Jul-2014 17:00 1.2M
![[TXT]](http://www.audentia-gestion.fr/icons/text.gif)
Farnell-LM19-Texas-I..> 18-Jul-2014 17:00 1.2M
![[TXT]](http://www.audentia-gestion.fr/icons/text.gif)
Farnell-Data-Sheet-S..> 18-Jul-2014 17:00 1.2M
![[TXT]](http://www.audentia-gestion.fr/icons/text.gif)
Farnell-LMH6518-Texa..> 18-Jul-2014 16:59 1.3M
![[TXT]](http://www.audentia-gestion.fr/icons/text.gif)
Farnell-AD7719-Low-V..> 18-Jul-2014 16:59 1.4M
![[TXT]](http://www.audentia-gestion.fr/icons/text.gif)
Farnell-DAC8143-Data..> 18-Jul-2014 16:59 1.5M
![[TXT]](http://www.audentia-gestion.fr/icons/text.gif)
Farnell-BGA7124-400-..> 18-Jul-2014 16:59 1.5M
![[TXT]](http://www.audentia-gestion.fr/icons/text.gif)
Farnell-SICK-OPTIC-E..> 18-Jul-2014 16:58 1.5M
![[TXT]](http://www.audentia-gestion.fr/icons/text.gif)
Farnell-LT3757-Linea..> 18-Jul-2014 16:58 1.6M
![[TXT]](http://www.audentia-gestion.fr/icons/text.gif)
Farnell-LT1961-Linea..> 18-Jul-2014 16:58 1.6M
![[TXT]](http://www.audentia-gestion.fr/icons/text.gif)
Farnell-PIC18F2420-2..> 18-Jul-2014 16:57 2.5M
![[TXT]](http://www.audentia-gestion.fr/icons/text.gif)
Farnell-DS3231-DS-PD..> 18-Jul-2014 16:57 2.5M
![[TXT]](http://www.audentia-gestion.fr/icons/text.gif)
Farnell-RDS-80-PDF.htm 18-Jul-2014 16:57 1.3M
![[TXT]](http://www.audentia-gestion.fr/icons/text.gif)
Farnell-AD8300-Data-..> 18-Jul-2014 16:56 1.3M
![[TXT]](http://www.audentia-gestion.fr/icons/text.gif)
Farnell-LT6233-Linea..> 18-Jul-2014 16:56 1.3M
![[TXT]](http://www.audentia-gestion.fr/icons/text.gif)
Farnell-MAX1365-MAX1..> 18-Jul-2014 16:56 1.4M
![[TXT]](http://www.audentia-gestion.fr/icons/text.gif)
Farnell-XPSAF5130-PD..> 18-Jul-2014 16:56 1.4M
![[TXT]](http://www.audentia-gestion.fr/icons/text.gif)
Farnell-DP83846A-DsP..> 18-Jul-2014 16:55 1.5M
![[TXT]](http://www.audentia-gestion.fr/icons/text.gif)
Farnell-Dremel-Exper..> 18-Jul-2014 16:55 1.6M
![[TXT]](http://www.audentia-gestion.fr/icons/text.gif)
Farnell-MCOC1-Farnel..> 16-Jul-2014 09:04 1.0M
![[TXT]](http://www.audentia-gestion.fr/icons/text.gif)
Farnell-SL3S1203_121..> 16-Jul-2014 09:04 1.1M
![[TXT]](http://www.audentia-gestion.fr/icons/text.gif)
Farnell-PN512-Full-N..> 16-Jul-2014 09:03 1.4M
![[TXT]](http://www.audentia-gestion.fr/icons/text.gif)
Farnell-SL3S4011_402..> 16-Jul-2014 09:03 1.1M
![[TXT]](http://www.audentia-gestion.fr/icons/text.gif)
Farnell-LPC408x-7x 3..> 16-Jul-2014 09:03 1.6M
![[TXT]](http://www.audentia-gestion.fr/icons/text.gif)
Farnell-PCF8574-PCF8..> 16-Jul-2014 09:03 1.7M
![[TXT]](http://www.audentia-gestion.fr/icons/text.gif)
Farnell-LPC81xM-32-b..> 16-Jul-2014 09:02 2.0M
![[TXT]](http://www.audentia-gestion.fr/icons/text.gif)
Farnell-LPC1769-68-6..> 16-Jul-2014 09:02 1.9M
![[TXT]](http://www.audentia-gestion.fr/icons/text.gif)
Farnell-Download-dat..> 16-Jul-2014 09:02 2.2M
![[TXT]](http://www.audentia-gestion.fr/icons/text.gif)
Farnell-LPC3220-30-4..> 16-Jul-2014 09:02 2.2M
![[TXT]](http://www.audentia-gestion.fr/icons/text.gif)
Farnell-LPC11U3x-32-..> 16-Jul-2014 09:01 2.4M
![[TXT]](http://www.audentia-gestion.fr/icons/text.gif)
Farnell-SL3ICS1002-1..> 16-Jul-2014 09:01 2.5M
![[TXT]](http://www.audentia-gestion.fr/icons/text.gif)
Farnell-T672-3000-Se..> 08-Jul-2014 18:59 2.0M
![[TXT]](http://www.audentia-gestion.fr/icons/text.gif)
Farnell-tesa®pack63..> 08-Jul-2014 18:56 2.0M
![[TXT]](http://www.audentia-gestion.fr/icons/text.gif)
Farnell-Encodeur-USB..> 08-Jul-2014 18:56 2.0M
![[TXT]](http://www.audentia-gestion.fr/icons/text.gif)
Farnell-CC2530ZDK-Us..> 08-Jul-2014 18:55 2.1M
![[TXT]](http://www.audentia-gestion.fr/icons/text.gif)
Farnell-2020-Manuel-..> 08-Jul-2014 18:55 2.1M
![[TXT]](http://www.audentia-gestion.fr/icons/text.gif)
Farnell-Synchronous-..> 08-Jul-2014 18:54 2.1M
![[TXT]](http://www.audentia-gestion.fr/icons/text.gif)
Farnell-Arithmetic-L..> 08-Jul-2014 18:54 2.1M
![[TXT]](http://www.audentia-gestion.fr/icons/text.gif)
Farnell-NA555-NE555-..> 08-Jul-2014 18:53 2.2M
![[TXT]](http://www.audentia-gestion.fr/icons/text.gif)
Farnell-4-Bit-Magnit..> 08-Jul-2014 18:53 2.2M
![[TXT]](http://www.audentia-gestion.fr/icons/text.gif)
Farnell-LM555-Timer-..> 08-Jul-2014 18:53 2.2M
![[TXT]](http://www.audentia-gestion.fr/icons/text.gif)
Farnell-L293d-Texas-..> 08-Jul-2014 18:53 2.2M
![[TXT]](http://www.audentia-gestion.fr/icons/text.gif)
Farnell-SN54HC244-SN..> 08-Jul-2014 18:52 2.3M
![[TXT]](http://www.audentia-gestion.fr/icons/text.gif)
Farnell-MAX232-MAX23..> 08-Jul-2014 18:52 2.3M
![[TXT]](http://www.audentia-gestion.fr/icons/text.gif)
Farnell-High-precisi..> 08-Jul-2014 18:51 2.3M
![[TXT]](http://www.audentia-gestion.fr/icons/text.gif)
Farnell-SMU-Instrume..> 08-Jul-2014 18:51 2.3M
![[TXT]](http://www.audentia-gestion.fr/icons/text.gif)
Farnell-900-Series-B..> 08-Jul-2014 18:50 2.3M
![[TXT]](http://www.audentia-gestion.fr/icons/text.gif)
Farnell-BA-Series-Oh..> 08-Jul-2014 18:50 2.3M
![[TXT]](http://www.audentia-gestion.fr/icons/text.gif)
Farnell-UTS-Series-S..> 08-Jul-2014 18:49 2.5M
![[TXT]](http://www.audentia-gestion.fr/icons/text.gif)
Farnell-270-Series-O..> 08-Jul-2014 18:49 2.3M
![[TXT]](http://www.audentia-gestion.fr/icons/text.gif)
Farnell-UTS-Series-S..> 08-Jul-2014 18:49 2.8M
![[TXT]](http://www.audentia-gestion.fr/icons/text.gif)
Farnell-Tiva-C-Serie..> 08-Jul-2014 18:49 2.6M
![[TXT]](http://www.audentia-gestion.fr/icons/text.gif)
Farnell-UTO-Souriau-..> 08-Jul-2014 18:48 2.8M
![[TXT]](http://www.audentia-gestion.fr/icons/text.gif)
Farnell-Clipper-Seri..> 08-Jul-2014 18:48 2.8M
![[TXT]](http://www.audentia-gestion.fr/icons/text.gif)
Farnell-SOURIAU-Cont..> 08-Jul-2014 18:47 3.0M
![[TXT]](http://www.audentia-gestion.fr/icons/text.gif)
Farnell-851-Series-P..> 08-Jul-2014 18:47 3.0M
Farnell-SL59830-Inte..> 06-Jul-2014 10:07 1.0M
![[TXT]](http://www.audentia-gestion.fr/icons/text.gif)
Farnell-ALF1210-PDF.htm 06-Jul-2014 10:06 4.0M
![[TXT]](http://www.audentia-gestion.fr/icons/text.gif)
Farnell-AD7171-16-Bi..> 06-Jul-2014 10:06 1.0M
![[TXT]](http://www.audentia-gestion.fr/icons/text.gif)
Farnell-Low-Noise-24..> 06-Jul-2014 10:05 1.0M
![[TXT]](http://www.audentia-gestion.fr/icons/text.gif)
Farnell-ESCON-Featur..> 06-Jul-2014 10:05 938K
![[TXT]](http://www.audentia-gestion.fr/icons/text.gif)
Farnell-74LCX573-Fai..> 06-Jul-2014 10:05 1.9M
![[TXT]](http://www.audentia-gestion.fr/icons/text.gif)
Farnell-1N4148WS-Fai..> 06-Jul-2014 10:04 1.9M
![[TXT]](http://www.audentia-gestion.fr/icons/text.gif)
Farnell-FAN6756-Fair..> 06-Jul-2014 10:04 850K
![[TXT]](http://www.audentia-gestion.fr/icons/text.gif)
Farnell-Datasheet-Fa..> 06-Jul-2014 10:04 861K
![[TXT]](http://www.audentia-gestion.fr/icons/text.gif)
Farnell-ES1F-ES1J-fi..> 06-Jul-2014 10:04 867K
![[TXT]](http://www.audentia-gestion.fr/icons/text.gif)
Farnell-QRE1113-Fair..> 06-Jul-2014 10:03 879K
![[TXT]](http://www.audentia-gestion.fr/icons/text.gif)
Farnell-2N7002DW-Fai..> 06-Jul-2014 10:03 886K
![[TXT]](http://www.audentia-gestion.fr/icons/text.gif)
Farnell-FDC2512-Fair..> 06-Jul-2014 10:03 886K
![[TXT]](http://www.audentia-gestion.fr/icons/text.gif)
Farnell-FDV301N-Digi..> 06-Jul-2014 10:03 886K
![[TXT]](http://www.audentia-gestion.fr/icons/text.gif)
Farnell-S1A-Fairchil..> 06-Jul-2014 10:03 896K
![[TXT]](http://www.audentia-gestion.fr/icons/text.gif)
Farnell-BAV99-Fairch..> 06-Jul-2014 10:03 896K
![[TXT]](http://www.audentia-gestion.fr/icons/text.gif)
Farnell-74AC00-74ACT..> 06-Jul-2014 10:03 911K
![[TXT]](http://www.audentia-gestion.fr/icons/text.gif)
Farnell-NaPiOn-Panas..> 06-Jul-2014 10:02 911K
![[TXT]](http://www.audentia-gestion.fr/icons/text.gif)
Farnell-LQ-RELAYS-AL..> 06-Jul-2014 10:02 924K
![[TXT]](http://www.audentia-gestion.fr/icons/text.gif)
Farnell-ev-relays-ae..> 06-Jul-2014 10:02 926K
![[TXT]](http://www.audentia-gestion.fr/icons/text.gif)
Farnell-ESCON-Featur..> 06-Jul-2014 10:02 931K
![[TXT]](http://www.audentia-gestion.fr/icons/text.gif)
Farnell-Amplifier-In..> 06-Jul-2014 10:02 940K
![[TXT]](http://www.audentia-gestion.fr/icons/text.gif)
Farnell-Serial-File-..> 06-Jul-2014 10:02 941K
![[TXT]](http://www.audentia-gestion.fr/icons/text.gif)
Farnell-Both-the-Del..> 06-Jul-2014 10:01 948K
![[TXT]](http://www.audentia-gestion.fr/icons/text.gif)
Farnell-Videk-PDF.htm 06-Jul-2014 10:01 948K
![[TXT]](http://www.audentia-gestion.fr/icons/text.gif)
Farnell-EPCOS-173438..> 04-Jul-2014 10:43 3.3M
![[TXT]](http://www.audentia-gestion.fr/icons/text.gif)
Farnell-Sensorless-C..> 04-Jul-2014 10:42 3.3M
![[TXT]](http://www.audentia-gestion.fr/icons/text.gif)
Farnell-197.31-KB-Te..> 04-Jul-2014 10:42 3.3M
![[TXT]](http://www.audentia-gestion.fr/icons/text.gif)
Farnell-PIC12F609-61..> 04-Jul-2014 10:41 3.7M
![[TXT]](http://www.audentia-gestion.fr/icons/text.gif)
Farnell-PADO-semi-au..> 04-Jul-2014 10:41 3.7M
![[TXT]](http://www.audentia-gestion.fr/icons/text.gif)
Farnell-03-iec-runds..> 04-Jul-2014 10:40 3.7M
![[TXT]](http://www.audentia-gestion.fr/icons/text.gif)
Farnell-ACC-Silicone..> 04-Jul-2014 10:40 3.7M
![[TXT]](http://www.audentia-gestion.fr/icons/text.gif)
Farnell-Series-TDS10..> 04-Jul-2014 10:39 4.0M
![[TXT]](http://www.audentia-gestion.fr/icons/text.gif)
Farnell-03-iec-runds..> 04-Jul-2014 10:40 3.7M
![[TXT]](http://www.audentia-gestion.fr/icons/text.gif)
Farnell-0430300011-D..> 14-Jun-2014 18:13 2.0M
![[TXT]](http://www.audentia-gestion.fr/icons/text.gif)
Farnell-06-6544-8-PD..> 26-Mar-2014 17:56 2.7M
![[TXT]](http://www.audentia-gestion.fr/icons/text.gif)
Farnell-3M-Polyimide..> 21-Mar-2014 08:09 3.9M
![[TXT]](http://www.audentia-gestion.fr/icons/text.gif)
Farnell-3M-VolitionT..> 25-Mar-2014 08:18 3.3M
![[TXT]](http://www.audentia-gestion.fr/icons/text.gif)
Farnell-10BQ060-PDF.htm 14-Jun-2014 09:50 2.4M
![[TXT]](http://www.audentia-gestion.fr/icons/text.gif)
Farnell-10TPB47M-End..> 14-Jun-2014 18:16 3.4M
![[TXT]](http://www.audentia-gestion.fr/icons/text.gif)
Farnell-12mm-Size-In..> 14-Jun-2014 09:50 2.4M
![[TXT]](http://www.audentia-gestion.fr/icons/text.gif)
Farnell-24AA024-24LC..> 23-Jun-2014 10:26 3.1M
![[TXT]](http://www.audentia-gestion.fr/icons/text.gif)
Farnell-50A-High-Pow..> 20-Mar-2014 17:31 2.9M
![[TXT]](http://www.audentia-gestion.fr/icons/text.gif)
Farnell-197.31-KB-Te..> 04-Jul-2014 10:42 3.3M
![[TXT]](http://www.audentia-gestion.fr/icons/text.gif)
Farnell-1907-2006-PD..> 26-Mar-2014 17:56 2.7M
![[TXT]](http://www.audentia-gestion.fr/icons/text.gif)
Farnell-5910-PDF.htm 25-Mar-2014 08:15 3.0M
![[TXT]](http://www.audentia-gestion.fr/icons/text.gif)
Farnell-6517b-Electr..> 29-Mar-2014 11:12 3.3M
![[TXT]](http://www.audentia-gestion.fr/icons/text.gif)
Farnell-A-True-Syste..> 29-Mar-2014 11:13 3.3M
![[TXT]](http://www.audentia-gestion.fr/icons/text.gif)
Farnell-ACC-Silicone..> 04-Jul-2014 10:40 3.7M
![[TXT]](http://www.audentia-gestion.fr/icons/text.gif)
Farnell-AD524-PDF.htm 20-Mar-2014 17:33 2.8M
![[TXT]](http://www.audentia-gestion.fr/icons/text.gif)
Farnell-ADL6507-PDF.htm 14-Jun-2014 18:19 3.4M
![[TXT]](http://www.audentia-gestion.fr/icons/text.gif)
Farnell-ADSP-21362-A..> 20-Mar-2014 17:34 2.8M
![[TXT]](http://www.audentia-gestion.fr/icons/text.gif)
Farnell-ALF1210-PDF.htm 04-Jul-2014 10:39 4.0M
![[TXT]](http://www.audentia-gestion.fr/icons/text.gif)
Farnell-ALF1225-12-V..> 01-Apr-2014 07:40 3.4M
![[TXT]](http://www.audentia-gestion.fr/icons/text.gif)
Farnell-ALF2412-24-V..> 01-Apr-2014 07:39 3.4M
![[TXT]](http://www.audentia-gestion.fr/icons/text.gif)
Farnell-AN10361-Phil..> 23-Jun-2014 10:29 2.1M
![[TXT]](http://www.audentia-gestion.fr/icons/text.gif)
Farnell-ARADUR-HY-13..> 26-Mar-2014 17:55 2.8M
![[TXT]](http://www.audentia-gestion.fr/icons/text.gif)
Farnell-ARALDITE-201..> 21-Mar-2014 08:12 3.7M
![[TXT]](http://www.audentia-gestion.fr/icons/text.gif)
Farnell-ARALDITE-CW-..> 26-Mar-2014 17:56 2.7M
![[TXT]](http://www.audentia-gestion.fr/icons/text.gif)
Farnell-ATMEL-8-bit-..> 19-Mar-2014 18:04 2.1M
![[TXT]](http://www.audentia-gestion.fr/icons/text.gif)
Farnell-ATMEL-8-bit-..> 11-Mar-2014 07:55 2.1M
![[TXT]](http://www.audentia-gestion.fr/icons/text.gif)
Farnell-ATmega640-VA..> 14-Jun-2014 09:49 2.5M
![[TXT]](http://www.audentia-gestion.fr/icons/text.gif)
Farnell-ATtiny20-PDF..> 25-Mar-2014 08:19 3.6M
![[TXT]](http://www.audentia-gestion.fr/icons/text.gif)
Farnell-ATtiny26-L-A..> 13-Jun-2014 18:40 1.8M
![[TXT]](http://www.audentia-gestion.fr/icons/text.gif)
Farnell-Alimentation..> 14-Jun-2014 18:24 2.5M
![[TXT]](http://www.audentia-gestion.fr/icons/text.gif)
Farnell-Alimentation..> 01-Apr-2014 07:42 3.4M
![[TXT]](http://www.audentia-gestion.fr/icons/text.gif)
Farnell-Amplificateu..> 29-Mar-2014 11:11 3.3M
![[TXT]](http://www.audentia-gestion.fr/icons/text.gif)
Farnell-An-Improved-..> 14-Jun-2014 09:49 2.5M
![[TXT]](http://www.audentia-gestion.fr/icons/text.gif)
Farnell-Atmel-ATmega..> 19-Mar-2014 18:03 2.2M
![[TXT]](http://www.audentia-gestion.fr/icons/text.gif)
Farnell-Avvertenze-e..> 14-Jun-2014 18:20 3.3M
![[TXT]](http://www.audentia-gestion.fr/icons/text.gif)
Farnell-BC846DS-NXP-..> 13-Jun-2014 18:42 1.6M
![[TXT]](http://www.audentia-gestion.fr/icons/text.gif)
Farnell-BC847DS-NXP-..> 23-Jun-2014 10:24 3.3M
![[TXT]](http://www.audentia-gestion.fr/icons/text.gif)
Farnell-BF545A-BF545..> 23-Jun-2014 10:28 2.1M
![[TXT]](http://www.audentia-gestion.fr/icons/text.gif)
Farnell-BK2650A-BK26..> 29-Mar-2014 11:10 3.3M
![[TXT]](http://www.audentia-gestion.fr/icons/text.gif)
Farnell-BT151-650R-N..> 13-Jun-2014 18:40 1.7M
![[TXT]](http://www.audentia-gestion.fr/icons/text.gif)
Farnell-BTA204-800C-..> 13-Jun-2014 18:42 1.6M
![[TXT]](http://www.audentia-gestion.fr/icons/text.gif)
Farnell-BUJD203AX-NX..> 13-Jun-2014 18:41 1.7M
![[TXT]](http://www.audentia-gestion.fr/icons/text.gif)
Farnell-BYV29F-600-N..> 13-Jun-2014 18:42 1.6M
![[TXT]](http://www.audentia-gestion.fr/icons/text.gif)
Farnell-BYV79E-serie..> 10-Mar-2014 16:19 1.6M
![[TXT]](http://www.audentia-gestion.fr/icons/text.gif)
Farnell-BZX384-serie..> 23-Jun-2014 10:29 2.1M
![[TXT]](http://www.audentia-gestion.fr/icons/text.gif)
Farnell-Battery-GBA-..> 14-Jun-2014 18:13 2.0M
![[TXT]](http://www.audentia-gestion.fr/icons/text.gif)
Farnell-C.A-6150-C.A..> 14-Jun-2014 18:24 2.5M
![[TXT]](http://www.audentia-gestion.fr/icons/text.gif)
Farnell-C.A 8332B-C...> 01-Apr-2014 07:40 3.4M
![[TXT]](http://www.audentia-gestion.fr/icons/text.gif)
Farnell-CC2560-Bluet..> 29-Mar-2014 11:14 2.8M
![[TXT]](http://www.audentia-gestion.fr/icons/text.gif)
Farnell-CD4536B-Type..> 14-Jun-2014 18:13 2.0M
![[TXT]](http://www.audentia-gestion.fr/icons/text.gif)
Farnell-CIRRUS-LOGIC..> 10-Mar-2014 17:20 2.1M
![[TXT]](http://www.audentia-gestion.fr/icons/text.gif)
Farnell-CS5532-34-BS..> 01-Apr-2014 07:39 3.5M
![[TXT]](http://www.audentia-gestion.fr/icons/text.gif)
Farnell-Cannon-ZD-PD..> 11-Mar-2014 08:13 2.8M
![[TXT]](http://www.audentia-gestion.fr/icons/text.gif)
Farnell-Ceramic-tran..> 14-Jun-2014 18:19 3.4M
![[TXT]](http://www.audentia-gestion.fr/icons/text.gif)
Farnell-Circuit-Note..> 26-Mar-2014 18:00 2.8M
![[TXT]](http://www.audentia-gestion.fr/icons/text.gif)
Farnell-Circuit-Note..> 26-Mar-2014 18:00 2.8M
![[TXT]](http://www.audentia-gestion.fr/icons/text.gif)
Farnell-Cles-electro..> 21-Mar-2014 08:13 3.9M
![[TXT]](http://www.audentia-gestion.fr/icons/text.gif)
Farnell-Conception-d..> 11-Mar-2014 07:49 2.4M
![[TXT]](http://www.audentia-gestion.fr/icons/text.gif)
Farnell-Connectors-N..> 14-Jun-2014 18:12 2.1M
![[TXT]](http://www.audentia-gestion.fr/icons/text.gif)
Farnell-Construction..> 14-Jun-2014 18:25 2.5M
![[TXT]](http://www.audentia-gestion.fr/icons/text.gif)
Farnell-Controle-de-..> 11-Mar-2014 08:16 2.8M
![[TXT]](http://www.audentia-gestion.fr/icons/text.gif)
Farnell-Cordless-dri..> 14-Jun-2014 18:13 2.0M
![[TXT]](http://www.audentia-gestion.fr/icons/text.gif)
Farnell-Current-Tran..> 26-Mar-2014 17:58 2.7M
![[TXT]](http://www.audentia-gestion.fr/icons/text.gif)
Farnell-Current-Tran..> 26-Mar-2014 17:58 2.7M
![[TXT]](http://www.audentia-gestion.fr/icons/text.gif)
Farnell-Current-Tran..> 26-Mar-2014 17:59 2.7M
![[TXT]](http://www.audentia-gestion.fr/icons/text.gif)
Farnell-Current-Tran..> 26-Mar-2014 17:59 2.7M
![[TXT]](http://www.audentia-gestion.fr/icons/text.gif)
Farnell-DC-Fan-type-..> 14-Jun-2014 09:48 2.5M
![[TXT]](http://www.audentia-gestion.fr/icons/text.gif)
Farnell-DC-Fan-type-..> 14-Jun-2014 09:51 1.8M
![[TXT]](http://www.audentia-gestion.fr/icons/text.gif)
Farnell-Davum-TMC-PD..> 14-Jun-2014 18:27 2.4M
![[TXT]](http://www.audentia-gestion.fr/icons/text.gif)
Farnell-De-la-puissa..> 29-Mar-2014 11:10 3.3M
![[TXT]](http://www.audentia-gestion.fr/icons/text.gif)
Farnell-Directive-re..> 25-Mar-2014 08:16 3.0M
![[TXT]](http://www.audentia-gestion.fr/icons/text.gif)
Farnell-Documentatio..> 14-Jun-2014 18:26 2.5M
![[TXT]](http://www.audentia-gestion.fr/icons/text.gif)
Farnell-Download-dat..> 13-Jun-2014 18:40 1.8M
![[TXT]](http://www.audentia-gestion.fr/icons/text.gif)
Farnell-ECO-Series-T..> 20-Mar-2014 08:14 2.5M
![[TXT]](http://www.audentia-gestion.fr/icons/text.gif)
Farnell-ELMA-PDF.htm 29-Mar-2014 11:13 3.3M
![[TXT]](http://www.audentia-gestion.fr/icons/text.gif)
Farnell-EMC1182-PDF.htm 25-Mar-2014 08:17 3.0M
![[TXT]](http://www.audentia-gestion.fr/icons/text.gif)
Farnell-EPCOS-173438..> 04-Jul-2014 10:43 3.3M
![[TXT]](http://www.audentia-gestion.fr/icons/text.gif)
Farnell-EPCOS-Sample..> 11-Mar-2014 07:53 2.2M
![[TXT]](http://www.audentia-gestion.fr/icons/text.gif)
Farnell-ES2333-PDF.htm 11-Mar-2014 08:14 2.8M
![[TXT]](http://www.audentia-gestion.fr/icons/text.gif)
Farnell-Ed.081002-DA..> 19-Mar-2014 18:02 2.5M
![[TXT]](http://www.audentia-gestion.fr/icons/text.gif)
Farnell-F28069-Picco..> 14-Jun-2014 18:14 2.0M
![[TXT]](http://www.audentia-gestion.fr/icons/text.gif)
Farnell-F42202-PDF.htm 19-Mar-2014 18:00 2.5M
![[TXT]](http://www.audentia-gestion.fr/icons/text.gif)
Farnell-FDS-ITW-Spra..> 14-Jun-2014 18:22 3.3M
![[TXT]](http://www.audentia-gestion.fr/icons/text.gif)
Farnell-FICHE-DE-DON..> 10-Mar-2014 16:17 1.6M
![[TXT]](http://www.audentia-gestion.fr/icons/text.gif)
Farnell-Fastrack-Sup..> 23-Jun-2014 10:25 3.3M
![[TXT]](http://www.audentia-gestion.fr/icons/text.gif)
Farnell-Ferric-Chlor..> 29-Mar-2014 11:14 2.8M
![[TXT]](http://www.audentia-gestion.fr/icons/text.gif)
Farnell-Fiche-de-don..> 14-Jun-2014 09:47 2.5M
![[TXT]](http://www.audentia-gestion.fr/icons/text.gif)
Farnell-Fiche-de-don..> 14-Jun-2014 18:26 2.5M
![[TXT]](http://www.audentia-gestion.fr/icons/text.gif)
Farnell-Fluke-1730-E..> 14-Jun-2014 18:23 2.5M
![[TXT]](http://www.audentia-gestion.fr/icons/text.gif)
Farnell-GALVA-A-FROI..> 26-Mar-2014 17:56 2.7M
![[TXT]](http://www.audentia-gestion.fr/icons/text.gif)
Farnell-GALVA-MAT-Re..> 26-Mar-2014 17:57 2.7M
![[TXT]](http://www.audentia-gestion.fr/icons/text.gif)
Farnell-GN-RELAYS-AG..> 20-Mar-2014 08:11 2.6M
![[TXT]](http://www.audentia-gestion.fr/icons/text.gif)
Farnell-HC49-4H-Crys..> 14-Jun-2014 18:20 3.3M
![[TXT]](http://www.audentia-gestion.fr/icons/text.gif)
Farnell-HFE1600-Data..> 14-Jun-2014 18:22 3.3M
![[TXT]](http://www.audentia-gestion.fr/icons/text.gif)
Farnell-HI-70300-Sol..> 14-Jun-2014 18:27 2.4M
![[TXT]](http://www.audentia-gestion.fr/icons/text.gif)
Farnell-HUNTSMAN-Adv..> 10-Mar-2014 16:17 1.7M
![[TXT]](http://www.audentia-gestion.fr/icons/text.gif)
Farnell-Haute-vitess..> 11-Mar-2014 08:17 2.4M
![[TXT]](http://www.audentia-gestion.fr/icons/text.gif)
Farnell-IP4252CZ16-8..> 13-Jun-2014 18:41 1.7M
![[TXT]](http://www.audentia-gestion.fr/icons/text.gif)
Farnell-Instructions..> 19-Mar-2014 18:01 2.5M
![[TXT]](http://www.audentia-gestion.fr/icons/text.gif)
Farnell-KSZ8851SNL-S..> 23-Jun-2014 10:28 2.1M
![[TXT]](http://www.audentia-gestion.fr/icons/text.gif)
Farnell-L-efficacite..> 11-Mar-2014 07:52 2.3M
![[TXT]](http://www.audentia-gestion.fr/icons/text.gif)
Farnell-LCW-CQ7P.CC-..> 25-Mar-2014 08:19 3.2M
![[TXT]](http://www.audentia-gestion.fr/icons/text.gif)
Farnell-LME49725-Pow..> 14-Jun-2014 09:49 2.5M
![[TXT]](http://www.audentia-gestion.fr/icons/text.gif)
Farnell-LOCTITE-542-..> 25-Mar-2014 08:15 3.0M
![[TXT]](http://www.audentia-gestion.fr/icons/text.gif)
Farnell-LOCTITE-3463..> 25-Mar-2014 08:19 3.0M
![[TXT]](http://www.audentia-gestion.fr/icons/text.gif)
Farnell-LUXEON-Guide..> 11-Mar-2014 07:52 2.3M
![[TXT]](http://www.audentia-gestion.fr/icons/text.gif)
Farnell-Leaded-Trans..> 23-Jun-2014 10:26 3.2M
![[TXT]](http://www.audentia-gestion.fr/icons/text.gif)
Farnell-Les-derniers..> 11-Mar-2014 07:50 2.3M
![[TXT]](http://www.audentia-gestion.fr/icons/text.gif)
Farnell-Loctite3455-..> 25-Mar-2014 08:16 3.0M
![[TXT]](http://www.audentia-gestion.fr/icons/text.gif)
Farnell-Low-cost-Enc..> 13-Jun-2014 18:42 1.7M
![[TXT]](http://www.audentia-gestion.fr/icons/text.gif)
Farnell-Lubrifiant-a..> 26-Mar-2014 18:00 2.7M
![[TXT]](http://www.audentia-gestion.fr/icons/text.gif)
Farnell-MC3510-PDF.htm 25-Mar-2014 08:17 3.0M
![[TXT]](http://www.audentia-gestion.fr/icons/text.gif)
Farnell-MC21605-PDF.htm 11-Mar-2014 08:14 2.8M
![[TXT]](http://www.audentia-gestion.fr/icons/text.gif)
Farnell-MCF532x-7x-E..> 29-Mar-2014 11:14 2.8M
![[TXT]](http://www.audentia-gestion.fr/icons/text.gif)
Farnell-MICREL-KSZ88..> 11-Mar-2014 07:54 2.2M
![[TXT]](http://www.audentia-gestion.fr/icons/text.gif)
Farnell-MICROCHIP-PI..> 19-Mar-2014 18:02 2.5M
![[TXT]](http://www.audentia-gestion.fr/icons/text.gif)
Farnell-MOLEX-39-00-..> 10-Mar-2014 17:19 1.9M
![[TXT]](http://www.audentia-gestion.fr/icons/text.gif)
Farnell-MOLEX-43020-..> 10-Mar-2014 17:21 1.9M
![[TXT]](http://www.audentia-gestion.fr/icons/text.gif)
Farnell-MOLEX-43160-..> 10-Mar-2014 17:21 1.9M
![[TXT]](http://www.audentia-gestion.fr/icons/text.gif)
Farnell-MOLEX-87439-..> 10-Mar-2014 17:21 1.9M
![[TXT]](http://www.audentia-gestion.fr/icons/text.gif)
Farnell-MPXV7002-Rev..> 20-Mar-2014 17:33 2.8M
![[TXT]](http://www.audentia-gestion.fr/icons/text.gif)
Farnell-MX670-MX675-..> 14-Jun-2014 09:46 2.5M
![[TXT]](http://www.audentia-gestion.fr/icons/text.gif)
Farnell-Microchip-MC..> 13-Jun-2014 18:27 1.8M
![[TXT]](http://www.audentia-gestion.fr/icons/text.gif)
Farnell-Microship-PI..> 11-Mar-2014 07:53 2.2M
![[TXT]](http://www.audentia-gestion.fr/icons/text.gif)
Farnell-Midas-Active..> 14-Jun-2014 18:17 3.4M
![[TXT]](http://www.audentia-gestion.fr/icons/text.gif)
Farnell-Midas-MCCOG4..> 14-Jun-2014 18:11 2.1M
![[TXT]](http://www.audentia-gestion.fr/icons/text.gif)
Farnell-Miniature-Ci..> 26-Mar-2014 17:55 2.8M
![[TXT]](http://www.audentia-gestion.fr/icons/text.gif)
Farnell-Mistral-PDF.htm 14-Jun-2014 18:12 2.1M
![[TXT]](http://www.audentia-gestion.fr/icons/text.gif)
Farnell-Molex-83421-..> 14-Jun-2014 18:17 3.4M
![[TXT]](http://www.audentia-gestion.fr/icons/text.gif)
Farnell-Molex-COMMER..> 14-Jun-2014 18:16 3.4M
![[TXT]](http://www.audentia-gestion.fr/icons/text.gif)
Farnell-Molex-Crimp-..> 10-Mar-2014 16:27 1.7M
![[TXT]](http://www.audentia-gestion.fr/icons/text.gif)
Farnell-Multi-Functi..> 20-Mar-2014 17:38 3.0M
![[TXT]](http://www.audentia-gestion.fr/icons/text.gif)
Farnell-NTE_SEMICOND..> 11-Mar-2014 07:52 2.3M
![[TXT]](http://www.audentia-gestion.fr/icons/text.gif)
Farnell-NXP-74VHC126..> 10-Mar-2014 16:17 1.6M
![[TXT]](http://www.audentia-gestion.fr/icons/text.gif)
Farnell-NXP-BT136-60..> 11-Mar-2014 07:52 2.3M
![[TXT]](http://www.audentia-gestion.fr/icons/text.gif)
Farnell-NXP-PBSS9110..> 10-Mar-2014 17:21 1.9M
![[TXT]](http://www.audentia-gestion.fr/icons/text.gif)
Farnell-NXP-PCA9555 ..> 11-Mar-2014 07:54 2.2M
![[TXT]](http://www.audentia-gestion.fr/icons/text.gif)
Farnell-NXP-PMBFJ620..> 10-Mar-2014 16:16 1.7M
![[TXT]](http://www.audentia-gestion.fr/icons/text.gif)
Farnell-NXP-PSMN1R7-..> 10-Mar-2014 16:17 1.6M
![[TXT]](http://www.audentia-gestion.fr/icons/text.gif)
Farnell-NXP-PSMN7R0-..> 10-Mar-2014 17:19 2.1M
![[TXT]](http://www.audentia-gestion.fr/icons/text.gif)
Farnell-NXP-TEA1703T..> 11-Mar-2014 08:15 2.8M
![[TXT]](http://www.audentia-gestion.fr/icons/text.gif)
Farnell-Nilï¬-sk-E-..> 14-Jun-2014 09:47 2.5M
![[TXT]](http://www.audentia-gestion.fr/icons/text.gif)
Farnell-Novembre-201..> 20-Mar-2014 17:38 3.3M
![[TXT]](http://www.audentia-gestion.fr/icons/text.gif)
Farnell-OMRON-Master..> 10-Mar-2014 16:26 1.8M
![[TXT]](http://www.audentia-gestion.fr/icons/text.gif)
Farnell-OSLON-SSL-Ce..> 19-Mar-2014 18:03 2.1M
![[TXT]](http://www.audentia-gestion.fr/icons/text.gif)
Farnell-OXPCIE958-FB..> 13-Jun-2014 18:40 1.8M
![[TXT]](http://www.audentia-gestion.fr/icons/text.gif)
Farnell-PADO-semi-au..> 04-Jul-2014 10:41 3.7M
![[TXT]](http://www.audentia-gestion.fr/icons/text.gif)
Farnell-PBSS5160T-60..> 19-Mar-2014 18:03 2.1M
![[TXT]](http://www.audentia-gestion.fr/icons/text.gif)
Farnell-PDTA143X-ser..> 20-Mar-2014 08:12 2.6M
![[TXT]](http://www.audentia-gestion.fr/icons/text.gif)
Farnell-PDTB123TT-NX..> 13-Jun-2014 18:43 1.5M
![[TXT]](http://www.audentia-gestion.fr/icons/text.gif)
Farnell-PESD5V0F1BL-..> 13-Jun-2014 18:43 1.5M
![[TXT]](http://www.audentia-gestion.fr/icons/text.gif)
Farnell-PESD9X5.0L-P..> 13-Jun-2014 18:43 1.6M
![[TXT]](http://www.audentia-gestion.fr/icons/text.gif)
Farnell-PIC12F609-61..> 04-Jul-2014 10:41 3.7M
![[TXT]](http://www.audentia-gestion.fr/icons/text.gif)
Farnell-PIC18F2455-2..> 23-Jun-2014 10:27 3.1M
![[TXT]](http://www.audentia-gestion.fr/icons/text.gif)
Farnell-PIC24FJ256GB..> 14-Jun-2014 09:51 2.4M
![[TXT]](http://www.audentia-gestion.fr/icons/text.gif)
Farnell-PMBT3906-PNP..> 13-Jun-2014 18:44 1.5M
![[TXT]](http://www.audentia-gestion.fr/icons/text.gif)
Farnell-PMBT4403-PNP..> 23-Jun-2014 10:27 3.1M
![[TXT]](http://www.audentia-gestion.fr/icons/text.gif)
Farnell-PMEG4002EL-N..> 14-Jun-2014 18:18 3.4M
![[TXT]](http://www.audentia-gestion.fr/icons/text.gif)
Farnell-PMEG4010CEH-..> 13-Jun-2014 18:43 1.6M
![[TXT]](http://www.audentia-gestion.fr/icons/text.gif)
Farnell-Panasonic-15..> 23-Jun-2014 10:29 2.1M
![[TXT]](http://www.audentia-gestion.fr/icons/text.gif)
Farnell-Panasonic-EC..> 20-Mar-2014 17:36 2.6M
![[TXT]](http://www.audentia-gestion.fr/icons/text.gif)
Farnell-Panasonic-EZ..> 20-Mar-2014 08:10 2.6M
![[TXT]](http://www.audentia-gestion.fr/icons/text.gif)
Farnell-Panasonic-Id..> 20-Mar-2014 17:35 2.6M
![[TXT]](http://www.audentia-gestion.fr/icons/text.gif)
Farnell-Panasonic-Ne..> 20-Mar-2014 17:36 2.6M
![[TXT]](http://www.audentia-gestion.fr/icons/text.gif)
Farnell-Panasonic-Ra..> 20-Mar-2014 17:37 2.6M
![[TXT]](http://www.audentia-gestion.fr/icons/text.gif)
Farnell-Panasonic-TS..> 20-Mar-2014 08:12 2.6M
![[TXT]](http://www.audentia-gestion.fr/icons/text.gif)
Farnell-Panasonic-Y3..> 20-Mar-2014 08:11 2.6M
![[TXT]](http://www.audentia-gestion.fr/icons/text.gif)
Farnell-Pico-Spox-Wi..> 10-Mar-2014 16:16 1.7M
![[TXT]](http://www.audentia-gestion.fr/icons/text.gif)
Farnell-Pompes-Charg..> 24-Apr-2014 20:23 3.3M
![[TXT]](http://www.audentia-gestion.fr/icons/text.gif)
Farnell-Ponts-RLC-po..> 14-Jun-2014 18:23 3.3M
![[TXT]](http://www.audentia-gestion.fr/icons/text.gif)
Farnell-Portable-Ana..> 29-Mar-2014 11:16 2.8M
![[TXT]](http://www.audentia-gestion.fr/icons/text.gif)
Farnell-Premier-Farn..> 21-Mar-2014 08:11 3.8M
![[TXT]](http://www.audentia-gestion.fr/icons/text.gif)
Farnell-Produit-3430..> 14-Jun-2014 09:48 2.5M
![[TXT]](http://www.audentia-gestion.fr/icons/text.gif)
Farnell-Proskit-SS-3..> 10-Mar-2014 16:26 1.8M
![[TXT]](http://www.audentia-gestion.fr/icons/text.gif)
Farnell-Puissance-ut..> 11-Mar-2014 07:49 2.4M
![[TXT]](http://www.audentia-gestion.fr/icons/text.gif)
Farnell-Q48-PDF.htm 23-Jun-2014 10:29 2.1M
![[TXT]](http://www.audentia-gestion.fr/icons/text.gif)
Farnell-Radial-Lead-..> 20-Mar-2014 08:12 2.6M
![[TXT]](http://www.audentia-gestion.fr/icons/text.gif)
Farnell-Realiser-un-..> 11-Mar-2014 07:51 2.3M
![[TXT]](http://www.audentia-gestion.fr/icons/text.gif)
Farnell-Reglement-RE..> 21-Mar-2014 08:08 3.9M
![[TXT]](http://www.audentia-gestion.fr/icons/text.gif)
Farnell-Repartiteurs..> 14-Jun-2014 18:26 2.5M
![[TXT]](http://www.audentia-gestion.fr/icons/text.gif)
Farnell-S-TRI-SWT860..> 21-Mar-2014 08:11 3.8M
![[TXT]](http://www.audentia-gestion.fr/icons/text.gif)
Farnell-SB175-Connec..> 11-Mar-2014 08:14 2.8M
![[TXT]](http://www.audentia-gestion.fr/icons/text.gif)
Farnell-SMBJ-Transil..> 29-Mar-2014 11:12 3.3M
![[TXT]](http://www.audentia-gestion.fr/icons/text.gif)
Farnell-SOT-23-Multi..> 11-Mar-2014 07:51 2.3M
![[TXT]](http://www.audentia-gestion.fr/icons/text.gif)
Farnell-SPLC780A1-16..> 14-Jun-2014 18:25 2.5M
![[TXT]](http://www.audentia-gestion.fr/icons/text.gif)
Farnell-SSC7102-Micr..> 23-Jun-2014 10:25 3.2M
![[TXT]](http://www.audentia-gestion.fr/icons/text.gif)
Farnell-SVPE-series-..> 14-Jun-2014 18:15 2.0M
![[TXT]](http://www.audentia-gestion.fr/icons/text.gif)
Farnell-Sensorless-C..> 04-Jul-2014 10:42 3.3M
![[TXT]](http://www.audentia-gestion.fr/icons/text.gif)
Farnell-Septembre-20..> 20-Mar-2014 17:46 3.7M
![[TXT]](http://www.audentia-gestion.fr/icons/text.gif)
Farnell-Serie-PicoSc..> 19-Mar-2014 18:01 2.5M
![[TXT]](http://www.audentia-gestion.fr/icons/text.gif)
Farnell-Serie-Standa..> 14-Jun-2014 18:23 3.3M
![[TXT]](http://www.audentia-gestion.fr/icons/text.gif)
Farnell-Series-2600B..> 20-Mar-2014 17:30 3.0M
![[TXT]](http://www.audentia-gestion.fr/icons/text.gif)
Farnell-Series-TDS10..> 04-Jul-2014 10:39 4.0M
![[TXT]](http://www.audentia-gestion.fr/icons/text.gif)
Farnell-Signal-PCB-R..> 14-Jun-2014 18:11 2.1M
![[TXT]](http://www.audentia-gestion.fr/icons/text.gif)
Farnell-Strangkuhlko..> 21-Mar-2014 08:09 3.9M
![[TXT]](http://www.audentia-gestion.fr/icons/text.gif)
Farnell-Supercapacit..> 26-Mar-2014 17:57 2.7M
![[TXT]](http://www.audentia-gestion.fr/icons/text.gif)
Farnell-TDK-Lambda-H..> 14-Jun-2014 18:21 3.3M
![[TXT]](http://www.audentia-gestion.fr/icons/text.gif)
Farnell-TEKTRONIX-DP..> 10-Mar-2014 17:20 2.0M
![[TXT]](http://www.audentia-gestion.fr/icons/text.gif)
Farnell-Tektronix-AC..> 13-Jun-2014 18:44 1.5M
![[TXT]](http://www.audentia-gestion.fr/icons/text.gif)
Farnell-Telemetres-l..> 20-Mar-2014 17:46 3.7M
![[TXT]](http://www.audentia-gestion.fr/icons/text.gif)
Farnell-Termometros-..> 14-Jun-2014 18:14 2.0M
![[TXT]](http://www.audentia-gestion.fr/icons/text.gif)
Farnell-The-essentia..> 10-Mar-2014 16:27 1.7M
![[TXT]](http://www.audentia-gestion.fr/icons/text.gif)
Farnell-U2270B-PDF.htm 14-Jun-2014 18:15 3.4M
![[TXT]](http://www.audentia-gestion.fr/icons/text.gif)
Farnell-USB-Buccanee..> 14-Jun-2014 09:48 2.5M
![[TXT]](http://www.audentia-gestion.fr/icons/text.gif)
Farnell-USB1T11A-PDF..> 19-Mar-2014 18:03 2.1M
![[TXT]](http://www.audentia-gestion.fr/icons/text.gif)
Farnell-V4N-PDF.htm 14-Jun-2014 18:11 2.1M
![[TXT]](http://www.audentia-gestion.fr/icons/text.gif)
Farnell-WetTantalum-..> 11-Mar-2014 08:14 2.8M
![[TXT]](http://www.audentia-gestion.fr/icons/text.gif)
Farnell-XPS-AC-Octop..> 14-Jun-2014 18:11 2.1M
![[TXT]](http://www.audentia-gestion.fr/icons/text.gif)
Farnell-XPS-MC16-XPS..> 11-Mar-2014 08:15 2.8M
![[TXT]](http://www.audentia-gestion.fr/icons/text.gif)
Farnell-YAGEO-DATA-S..> 11-Mar-2014 08:13 2.8M
![[TXT]](http://www.audentia-gestion.fr/icons/text.gif)
Farnell-ZigBee-ou-le..> 11-Mar-2014 07:50 2.4M
![[TXT]](http://www.audentia-gestion.fr/icons/text.gif)
Farnell-celpac-SUL84..> 21-Mar-2014 08:11 3.8M
![[TXT]](http://www.audentia-gestion.fr/icons/text.gif)
Farnell-china_rohs_o..> 21-Mar-2014 10:04 3.9M
![[TXT]](http://www.audentia-gestion.fr/icons/text.gif)
Farnell-cree-Xlamp-X..> 20-Mar-2014 17:34 2.8M
![[TXT]](http://www.audentia-gestion.fr/icons/text.gif)
Farnell-cree-Xlamp-X..> 20-Mar-2014 17:35 2.7M
![[TXT]](http://www.audentia-gestion.fr/icons/text.gif)
Farnell-cree-Xlamp-X..> 20-Mar-2014 17:31 2.9M
![[TXT]](http://www.audentia-gestion.fr/icons/text.gif)
Farnell-cree-Xlamp-m..> 20-Mar-2014 17:32 2.9M
![[TXT]](http://www.audentia-gestion.fr/icons/text.gif)
Farnell-cree-Xlamp-m..> 20-Mar-2014 17:32 2.9M
![[TXT]](http://www.audentia-gestion.fr/icons/text.gif)
Farnell-ir1150s_fr.p..> 29-Mar-2014 11:11 3.3M
![[TXT]](http://www.audentia-gestion.fr/icons/text.gif)
Farnell-manual-bus-p..> 10-Mar-2014 16:29 1.9M
![[TXT]](http://www.audentia-gestion.fr/icons/text.gif)
Farnell-propose-plus..> 11-Mar-2014 08:19 2.8M
![[TXT]](http://www.audentia-gestion.fr/icons/text.gif)
Farnell-techfirst_se..> 21-Mar-2014 08:08 3.9M
![[TXT]](http://www.audentia-gestion.fr/icons/text.gif)
Farnell-testo-205-20..> 20-Mar-2014 17:37 3.0M
![[TXT]](http://www.audentia-gestion.fr/icons/text.gif)
Farnell-testo-470-Fo..> 20-Mar-2014 17:38 3.0M
![[TXT]](http://www.audentia-gestion.fr/icons/text.gif)
Farnell-uC-OS-III-Br..> 10-Mar-2014 17:20 2.0M
![[TXT]](http://www.audentia-gestion.fr/icons/text.gif)
Sefram-7866HD.pdf-PD..> 29-Mar-2014 11:46 472K
![[TXT]](http://www.audentia-gestion.fr/icons/text.gif)
Sefram-CAT_ENREGISTR..> 29-Mar-2014 11:46 461K
![[TXT]](http://www.audentia-gestion.fr/icons/text.gif)
Sefram-CAT_MESUREURS..> 29-Mar-2014 11:46 435K
![[TXT]](http://www.audentia-gestion.fr/icons/text.gif)
Sefram-GUIDE_SIMPLIF..> 29-Mar-2014 11:46 481K
![[TXT]](http://www.audentia-gestion.fr/icons/text.gif)
Sefram-GUIDE_SIMPLIF..> 29-Mar-2014 11:46 442K
![[TXT]](http://www.audentia-gestion.fr/icons/text.gif)
Sefram-GUIDE_SIMPLIF..> 29-Mar-2014 11:46 422K
![[TXT]](http://www.audentia-gestion.fr/icons/text.gif)
Sefram-SP270.pdf-PDF..> 29-Mar-2014 11:46 464K
1
2
3
4
5
6
7
8
9
18
17
16
15
14
13
12
11
10
1B
2B
3B
4B
5B
6B
7B
8B
GND
1C
2C
3C
4C
5C
6C
7C
8C
COM
DW OR N PACKAGE
(TOP VIEW)
ULN2803A
www.ti.com SLRS049F –FEBRUARY 1997–REVISED JANUARY 2014
ULN2803A Darlington Transistor Arrays
Check for Samples: ULN2803A
1FEATURES DESCRIPTION
• 500-mA-Rated Collector Current The ULN2803A device is a high-voltage, high-current
(Single Output) Darlington transistor array. The device consists of
eight npn Darlington pairs that feature high-voltage
• High-Voltage Outputs: 50 V outputs with common-cathode clamp diodes for
• Output Clamp Diodes switching inductive loads. The collector-current rating
• Inputs Compatible With Various of each Darlington pair is 500 mA. The Darlington
Types of Logic pairs may be connected in parallel for higher current capability.
• Relay-Driver Applications
• Compatible with ULN2800A Series Applications include relay drivers, hammer drivers, lamp drivers, display drivers (LED and gas
discharge), line drivers, and logic buffers. The
ULN2803A device has a 2.7-kΩ series base resistor
for each Darlington pair for operation directly with
TTL or 5-V CMOS devices.
1
Please be aware that an important notice concerning availability, standard warranty, and use in critical applications of
Texas Instruments semiconductor products and disclaimers thereto appears at the end of this data sheet.
PRODUCTION DATA information is current as of publication date. Copyright © 1997–2014, Texas Instruments Incorporated Products conform to specifications per the terms of the Texas
Instruments standard warranty. Production processing does not
necessarily include testing of all parameters.
2.7 k!
7.2 k! 3 k!
COM
Output C
E
Input B
8C
7C
6C
5C
4C
3C
2C
7
6
5
4
3
2
1
7B
6B
5B
4B
3B
2B
1B
11
12
13
14
15
16
17
COM
8
8B
10
1C
18
ULN2803A
SLRS049F –FEBRUARY 1997–REVISED JANUARY 2014 www.ti.com
This integrated circuit can be damaged by ESD. Texas Instruments recommends that all integrated circuits be handled with
appropriate precautions. Failure to observe proper handling and installation procedures can cause damage.
ESD damage can range from subtle performance degradation to complete device failure. Precision integrated circuits may be more
susceptible to damage because very small parametric changes could cause the device not to meet its published specifications.
Logic Diagram
Schematic (Each Darlington Pair)
2 Submit Documentation Feedback Copyright © 1997–2014, Texas Instruments Incorporated
Product Folder Links :ULN2803A
ULN2803A
www.ti.com SLRS049F –FEBRUARY 1997–REVISED JANUARY 2014
Absolute Maximum Ratings(1)
at 25°C free-air temperature (unless otherwise noted)
VALUE UNIT
Collector-emitter voltage 50 V
Input voltage(2) 30 V
Peak collector current 500 mA
Output clamp current 500 mA
Total substrate-terminal current –2.5 A
D package 73.14
θJA Package thermal impedance(3) (4) °C/W
DW package 62.66
TJ Operating virtual junction temperature 150 °C
Tstg Storage temperature range –65 to 150 °C
(1) Stresses beyond those listed under Absolute Maximum Ratings may cause permanent damage to the device. These are stress ratings
only, and functional operation of the device at these or any other conditions beyond those indicated under Recommended Operating
Conditions is not implied. Exposure to absolute-maximum-rated conditions for extended periods may affect device reliability.
(2) All voltage values, unless otherwise noted, are with respect to the emitter/substrate terminal GND.
(3) Maximum power dissipation is a function of TJ(max), θJA, and TA. The maximum allowable power dissipation at any allowable ambient
temperature is PD = (TJ(max) – TA)/θJA. Operating at the absolute maximum TJ of 150°C can affect reliability.
(4) The package thermal impedance is calculated in accordance with JESD 51-7.
Electrical Characteristics
at TA = 25°C free-air temperature (unless otherwise noted)
ULN2002A
PARAMETER TEST CONDITIONS UNIT
MIN TYP MAX
I VCE = 50 V, CEX Collector cutoff current see Figure 1 II = 0 50 μA
I VCE = 50 V, IC = 500 μA, I(off) Off-state input current T 50 65 μA A = 70°C see Figure 2
II(on) Input current VI = 3.85 V, See Figure 3 0.93 1.35 mA
IC = 200 mA 2.4
V VCE = 2 V, I(on) On-state input voltage see Figure 4 IC = 250 mA 2.7 V
IC = 300 mA 3
II = 250 μA, IC = 100 mA 0.9 1.1 see Figure 5
V II = 350 μA, CE(sat) Collector-emitter saturation voltage see Figure 5 IC = 200 mA 1 1.3 V
II = 500 μA, IC = 350 mA 1.3 1.6 see Figure 5
IR Clamp diode reverse current VR = 50 V, see Figure 6 50 μA
VF Clamp diode forward voltage IF = 350 mA see Figure 7 1.7 2 V
Ci Input capacitance VI = 0, f = 1 MHz 15 25 pF
Switching Characteristics
TA = 25°C
PARAMETER TEST CONDITIONS MIN TYP MAX UNIT
tPLH Propagation delay time, low- to high-level output VS = 50 V, CL = 15 pF, RL = 163 Ω, 130 See Figure 8 ns tPHL Propagation delay time, high- to low-level output 20
VOH High-level output voltage after switching VS = 50 V, IO = 300 mA, See Figure 9 VS – 20 mV
Copyright © 1997–2014, Texas Instruments Incorporated Submit Documentation Feedback 3
Product Folder Links :ULN2803A
Open
VF
IF
Open
VCE
II IC
hFE =
IC
II
VR
Open
IR
Open
VCE
IC
VI
Open
II
VI Open
Open VCE
IC
II(off)
Open VCE
ICEX
Open
ULN2803A
SLRS049F –FEBRUARY 1997–REVISED JANUARY 2014 www.ti.com
Parameter Measurement Information
Figure 1. ICEX Test Circuit Figure 2. II(off) Test Circuit
Figure 3. II(on) Test Circuit Figure 4. VI(on) Test Circuit
Figure 5. hFE, VCE(sat) Test Circuit Figure 6. IR Test Circuit
Figure 7. VF Test Circuit
4 Submit Documentation Feedback Copyright © 1997–2014, Texas Instruments Incorporated
Product Folder Links :ULN2803A
Pulse
Generator
(see Note A)
Input Open VS = 50 V
RL = 163 !
CL = 15 pF
(see Note B)
Output
tPHL tPLH
0.5 μs
<5 ns <10 ns
90%
50%
10% 10%
90%
50%
50% 50%
VIH
Input (see Note C)
Output
0
Test Circuit
Voltage Waveforms
VOH
ULN2803A
www.ti.com SLRS049F –FEBRUARY 1997–REVISED JANUARY 2014
Parameter Measurement Information (continued)
A. The pulse generator has the following characteristics: PRR = 12.5 kHz, ZO = 50 Ω.
B. CL includes probe and jig capacitance.
C. VIH = 3 V
Figure 8. Propagation Delay-Times
Copyright © 1997–2014, Texas Instruments Incorporated Submit Documentation Feedback 5
Product Folder Links :ULN2803A
Pulse
Generator
(see Note A)
Input
VS
163 !
CL = 15 pF
(see Note B)
Output
40 μs
<5 ns <10 ns
90%
1.5 V
10% 10%
90%
1.5 V
VIH
Input (see Note C)
Output
0
2 mH
VOH
Test Circuit
Voltage Waveforms
ULN2803A
SLRS049F –FEBRUARY 1997–REVISED JANUARY 2014 www.ti.com
Parameter Measurement Information (continued)
A. The pulse generator has the following characteristics: PRR = 12.5 kHz, ZO = 50 Ω.
B. CL includes probe and jig capacitance.
C. VIH = 3 V
Figure 9. Latch-Up Test
6 Submit Documentation Feedback Copyright © 1997–2014, Texas Instruments Incorporated
Product Folder Links :ULN2803A
ULN2803A
www.ti.com SLRS049F –FEBRUARY 1997–REVISED JANUARY 2014
REVISION HISTORY
Changes from Revision E (July 2006) to Revision F Page
• Updated document to new TI data sheet format - no specification changes. ...................................................................... 1
• Deleted Ordering Information table. ...................................................................................................................................... 1
• Added ESD warning. ............................................................................................................................................................ 2
Copyright © 1997–2014, Texas Instruments Incorporated Submit Documentation Feedback 7
Product Folder Links :ULN2803A
PACKAGE OPTION ADDENDUM
www.ti.com 27-Jan-2014
Addendum-Page 1
PACKAGING INFORMATION
Orderable Device Status
(1)
Package Type Package
Drawing
Pins Package
Qty
Eco Plan
(2)
Lead/Ball Finish
(6)
MSL Peak Temp
(3)
Op Temp (°C) Device Marking
(4/5)
Samples
ULN2803ADW ACTIVE SOIC DW 18 40 Green (RoHS
& no Sb/Br)
CU NIPDAU Level-2-260C-1 YEAR -40 to 85 ULN2803A
ULN2803ADWG4 ACTIVE SOIC DW 18 40 Green (RoHS
& no Sb/Br)
CU NIPDAU Level-2-260C-1 YEAR -40 to 85 ULN2803A
ULN2803ADWR ACTIVE SOIC DW 18 2000 Green (RoHS
& no Sb/Br)
CU NIPDAU Level-2-260C-1 YEAR -40 to 85 ULN2803A
ULN2803ADWRG4 ACTIVE SOIC DW 18 2000 Green (RoHS
& no Sb/Br)
CU NIPDAU Level-2-260C-1 YEAR -40 to 85 ULN2803A
ULN2803AN ACTIVE PDIP N 18 20 Pb-Free
(RoHS)
CU NIPDAU N / A for Pkg Type -40 to 85 ULN2803AN
ULN2803ANE4 ACTIVE PDIP N 18 20 Pb-Free
(RoHS)
CU NIPDAU N / A for Pkg Type -40 to 85 ULN2803AN
(1) The marketing status values are defined as follows:
ACTIVE: Product device recommended for new designs.
LIFEBUY: TI has announced that the device will be discontinued, and a lifetime-buy period is in effect.
NRND: Not recommended for new designs. Device is in production to support existing customers, but TI does not recommend using this part in a new design.
PREVIEW: Device has been announced but is not in production. Samples may or may not be available.
OBSOLETE: TI has discontinued the production of the device.
(2) Eco Plan - The planned eco-friendly classification: Pb-Free (RoHS), Pb-Free (RoHS Exempt), or Green (RoHS & no Sb/Br) - please check http://www.ti.com/productcontent for the latest availability
information and additional product content details.
TBD: The Pb-Free/Green conversion plan has not been defined.
Pb-Free (RoHS): TI's terms "Lead-Free" or "Pb-Free" mean semiconductor products that are compatible with the current RoHS requirements for all 6 substances, including the requirement that
lead not exceed 0.1% by weight in homogeneous materials. Where designed to be soldered at high temperatures, TI Pb-Free products are suitable for use in specified lead-free processes.
Pb-Free (RoHS Exempt): This component has a RoHS exemption for either 1) lead-based flip-chip solder bumps used between the die and package, or 2) lead-based die adhesive used between
the die and leadframe. The component is otherwise considered Pb-Free (RoHS compatible) as defined above.
Green (RoHS & no Sb/Br): TI defines "Green" to mean Pb-Free (RoHS compatible), and free of Bromine (Br) and Antimony (Sb) based flame retardants (Br or Sb do not exceed 0.1% by weight
in homogeneous material)
(3) MSL, Peak Temp. - The Moisture Sensitivity Level rating according to the JEDEC industry standard classifications, and peak solder temperature.
(4) There may be additional marking, which relates to the logo, the lot trace code information, or the environmental category on the device.
(5) Multiple Device Markings will be inside parentheses. Only one Device Marking contained in parentheses and separated by a "~" will appear on a device. If a line is indented then it is a continuation
of the previous line and the two combined represent the entire Device Marking for that device.
PACKAGE OPTION ADDENDUM
www.ti.com 27-Jan-2014
Addendum-Page 2
(6) Lead/Ball Finish - Orderable Devices may have multiple material finish options. Finish options are separated by a vertical ruled line. Lead/Ball Finish values may wrap to two lines if the finish
value exceeds the maximum column width.
Important Information and Disclaimer:The information provided on this page represents TI's knowledge and belief as of the date that it is provided. TI bases its knowledge and belief on information
provided by third parties, and makes no representation or warranty as to the accuracy of such information. Efforts are underway to better integrate information from third parties. TI has taken and
continues to take reasonable steps to provide representative and accurate information but may not have conducted destructive testing or chemical analysis on incoming materials and chemicals.
TI and TI suppliers consider certain information to be proprietary, and thus CAS numbers and other limited information may not be available for release.
In no event shall TI's liability arising out of such information exceed the total purchase price of the TI part(s) at issue in this document sold by TI to Customer on an annual basis.
TAPE AND REEL INFORMATION
*All dimensions are nominal
Device Package
Type
Package
Drawing
Pins SPQ Reel
Diameter
(mm)
Reel
Width
W1 (mm)
A0
(mm)
B0
(mm)
K0
(mm)
P1
(mm)
W
(mm)
Pin1
Quadrant
ULN2803ADWR SOIC DW 18 2000 330.0 24.4 10.9 12.0 2.7 12.0 24.0 Q1
PACKAGE MATERIALS INFORMATION
www.ti.com 27-Jan-2014
Pack Materials-Page 1
*All dimensions are nominal
Device Package Type Package Drawing Pins SPQ Length (mm) Width (mm) Height (mm)
ULN2803ADWR SOIC DW 18 2000 370.0 355.0 55.0
PACKAGE MATERIALS INFORMATION
www.ti.com 27-Jan-2014
Pack Materials-Page 2
IMPORTANT NOTICE
Texas Instruments Incorporated and its subsidiaries (TI) reserve the right to make corrections, enhancements, improvements and other
changes to its semiconductor products and services per JESD46, latest issue, and to discontinue any product or service per JESD48, latest
issue. Buyers should obtain the latest relevant information before placing orders and should verify that such information is current and
complete. All semiconductor products (also referred to herein as “components”) are sold subject to TI’s terms and conditions of sale
supplied at the time of order acknowledgment.
TI warrants performance of its components to the specifications applicable at the time of sale, in accordance with the warranty in TI’s terms
and conditions of sale of semiconductor products. Testing and other quality control techniques are used to the extent TI deems necessary
to support this warranty. Except where mandated by applicable law, testing of all parameters of each component is not necessarily
performed.
TI assumes no liability for applications assistance or the design of Buyers’ products. Buyers are responsible for their products and
applications using TI components. To minimize the risks associated with Buyers’ products and applications, Buyers should provide
adequate design and operating safeguards.
TI does not warrant or represent that any license, either express or implied, is granted under any patent right, copyright, mask work right, or
other intellectual property right relating to any combination, machine, or process in which TI components or services are used. Information
published by TI regarding third-party products or services does not constitute a license to use such products or services or a warranty or
endorsement thereof. Use of such information may require a license from a third party under the patents or other intellectual property of the
third party, or a license from TI under the patents or other intellectual property of TI.
Reproduction of significant portions of TI information in TI data books or data sheets is permissible only if reproduction is without alteration
and is accompanied by all associated warranties, conditions, limitations, and notices. TI is not responsible or liable for such altered
documentation. Information of third parties may be subject to additional restrictions.
Resale of TI components or services with statements different from or beyond the parameters stated by TI for that component or service
voids all express and any implied warranties for the associated TI component or service and is an unfair and deceptive business practice.
TI is not responsible or liable for any such statements.
Buyer acknowledges and agrees that it is solely responsible for compliance with all legal, regulatory and safety-related requirements
concerning its products, and any use of TI components in its applications, notwithstanding any applications-related information or support
that may be provided by TI. Buyer represents and agrees that it has all the necessary expertise to create and implement safeguards which
anticipate dangerous consequences of failures, monitor failures and their consequences, lessen the likelihood of failures that might cause
harm and take appropriate remedial actions. Buyer will fully indemnify TI and its representatives against any damages arising out of the use
of any TI components in safety-critical applications.
In some cases, TI components may be promoted specifically to facilitate safety-related applications. With such components, TI’s goal is to
help enable customers to design and create their own end-product solutions that meet applicable functional safety standards and
requirements. Nonetheless, such components are subject to these terms.
No TI components are authorized for use in FDA Class III (or similar life-critical medical equipment) unless authorized officers of the parties
have executed a special agreement specifically governing such use.
Only those TI components which TI has specifically designated as military grade or “enhanced plastic” are designed and intended for use in
military/aerospace applications or environments. Buyer acknowledges and agrees that any military or aerospace use of TI components
which have not been so designated is solely at the Buyer's risk, and that Buyer is solely responsible for compliance with all legal and
regulatory requirements in connection with such use.
TI has specifically designated certain components as meeting ISO/TS16949 requirements, mainly for automotive use. In any case of use of
non-designated products, TI will not be responsible for any failure to meet ISO/TS16949.
Products Applications
Audio www.ti.com/audio Automotive and Transportation www.ti.com/automotive
Amplifiers amplifier.ti.com Communications and Telecom www.ti.com/communications
Data Converters dataconverter.ti.com Computers and Peripherals www.ti.com/computers
DLP® Products www.dlp.com Consumer Electronics www.ti.com/consumer-apps
DSP dsp.ti.com Energy and Lighting www.ti.com/energy
Clocks and Timers www.ti.com/clocks Industrial www.ti.com/industrial
Interface interface.ti.com Medical www.ti.com/medical
Logic logic.ti.com Security www.ti.com/security
Power Mgmt power.ti.com Space, Avionics and Defense www.ti.com/space-avionics-defense
Microcontrollers microcontroller.ti.com Video and Imaging www.ti.com/video
RFID www.ti-rfid.com
OMAP Applications Processors www.ti.com/omap TI E2E Community e2e.ti.com
Wireless Connectivity www.ti.com/wirelessconnectivity
Mailing Address: Texas Instruments, Post Office Box 655303, Dallas, Texas 75265
Copyright © 2014, Texas Instruments Incorporated
MAX3222/MAX3232/MAX3237/MAX3241*
3.0V to 5.5V, Low-Power, up to 1Mbps, True RS-232
Transceivers Using Four 0.1μF External Capacitors
________________________________________________________________ Maxim Integrated Products 1
19-0273; Rev 7; 1/07
For pricing, delivery, and ordering information, please contact Maxim/Dallas Direct! at
1-888-629-4642, or visit Maxim’s website at www.maxim-ic.com.
________________General Description
The MAX3222/MAX3232/MAX3237/MAX3241 transceivers
have a proprietary low-dropout transmitter output
stage enabling true RS-232 performance from a
3.0V to 5.5V supply with a dual charge pump. The
devices require only four small 0.1μF external chargepump
capacitors. The MAX3222, MAX3232, and
MAX3241 are guaranteed to run at data rates of
120kbps while maintaining RS-232 output levels. The
MAX3237 is guaranteed to run at data rates of 250kbps
in the normal operating mode and 1Mbps in the
MegaBaud™ operating mode, while maintaining RS-232
output levels.
The MAX3222/MAX3232 have 2 receivers and 2
drivers. The MAX3222 features a 1μA shutdown mode
that reduces power consumption and extends battery
life in portable systems. Its receivers remain active in
shutdown mode, allowing external devices such as
modems to be monitored using only 1μA supply current.
The MAX3222 and MAX3232 are pin, package,
and functionally compatible with the industry-standard
MAX242 and MAX232, respectively.
The MAX3241 is a complete serial port (3 drivers/
5 receivers) designed for notebook and subnotebook
computers. The MAX3237 (5 drivers/3 receivers) is ideal
for fast modem applications. Both these devices feature
a shutdown mode in which all receivers can remain
active while using only 1μA supply current. Receivers R1
(MAX3237/MAX3241) and R2 (MAX3241) have extra outputs
in addition to their standard outputs. These extra
outputs are always active, allowing external devices
such as a modem to be monitored without forward biasing
the protection diodes in circuitry that may have VCC
completely removed.
The MAX3222, MAX3232, and MAX3241 are available
in space-saving TSSOP and SSOP packages.
________________________Applications
Notebook, Subnotebook, and Palmtop Computers
High-Speed Modems
Battery-Powered Equipment
Hand-Held Equipment
Peripherals
Printers
__Next Generation Device Features
♦ For Smaller Packaging:
MAX3228E/MAX3229E: +2.5V to +5.5V RS-232
Transceivers in UCSP™
♦ For Integrated ESD Protection:
MAX3222E/MAX3232E/MAX3237E/MAX3241E*/
MAX3246E: ±15kV ESD-Protected, Down to 10nA,
3.0V to 5.5V, Up to 1Mbps, True RS-232
Transceivers
♦ For Low-Voltage or Data Cable Applications:
MAX3380E/MAX3381E: +2.35V to +5.5V, 1μA,
2Tx/2Rx RS-232 Transceivers with ±15kV
ESD-Protected I/O and Logic Pins
18
17
16
15
14
13
12
11
1
2
3
4
5
6
7
8
SHDN
VCC
GND
C1- T1OUT
V+
C1+
EN
TOP VIEW
R1IN
R1OUT
T1IN
T2OUT T2IN
VC2-
C2+
R2IN 9 10 R2OUT
DIP/SO
MAX3222
+
MAX3222
_________________Pin Configurations
_______________Ordering Information
MegaBaud and UCSP are trademarks of Maxim Integrated Products, Inc.
*Covered by U.S. Patent numbers 4,636,930; 4,679,134; 4,777,577; 4,797,899; 4,809,152; 4,897,774; 4,999,761; and other patents pending.
Typical Operating Circuits appear at end of data sheet.
Pin Configurations continued at end of data sheet.
Ordering Information continued at end of data sheet.
+Denotes lead-free package.
PART TEMP RANGE PIN-PACKAGE
PKG
CODE
MAX3222CUP+ 0°C to +70°C 20 TSSOP U20+2
MAX3222CAP+ 0°C to +70°C 20 SSOP A20+1
MAX3222CWN+ 0°C to +70°C 18 SO W18+1
MAX3222CPN+ 0°C to +70°C 18 Plastic Dip P18+5
VCC = 5.0V
MAX3222/MAX3232/MAX3237/MAX3241
3.0V to 5.5V, Low-Power, up to 1Mbps, True RS-232
Transceivers Using Four 0.1μF External Capacitors
2 _______________________________________________________________________________________
ABSOLUTE MAXIMUM RATINGS
ELECTRICAL CHARACTERISTICS
(VCC = +3.0V to +5.5V, C1–C4 = 0.1μF (Note 2), TA = TMIN to TMAX, unless otherwise noted. Typical values are at TA = +25°C.)
Stresses beyond those listed under “Absolute Maximum Ratings” may cause permanent damage to the device. These are stress ratings only, and functional
operation of the device at these or any other conditions beyond those indicated in the operational sections of the specifications is not implied. Exposure to
absolute maximum rating conditions for extended periods may affect device reliability.
Note 1: V+ and V- can have a maximum magnitude of 7V, but their absolute difference cannot exceed 13V.
VCC...........................................................................-0.3V to +6V
V+ (Note 1) ...............................................................-0.3V to +7V
V- (Note 1) ................................................................+0.3V to -7V
V+ + V- (Note 1)...................................................................+13V
Input Voltages
T_IN, SHDN, EN ...................................................-0.3V to +6V
MBAUD...................................................-0.3V to (VCC + 0.3V)
R_IN .................................................................................±25V
Output Voltages
T_OUT...........................................................................±13.2V
R_OUT....................................................-0.3V to (VCC + 0.3V)
Short-Circuit Duration
T_OUT ....................................................................Continuous
Continuous Power Dissipation (TA = +70°C)
16-Pin TSSOP (derate 6.7mW/°C above +70°C).............533mW
16-Pin Narrow SO (derate 8.70mW/°C above +70°C) ....696mW
16-Pin Wide SO (derate 9.52mW/°C above +70°C)........762mW
16-Pin Plastic DIP (derate 10.53mW/°C above +70°C)...842mW
18-Pin SO (derate 9.52mW/°C above +70°C)..............762mW
18-Pin Plastic DIP (derate 11.11mW/°C above +70°C) ..889mW
20-Pin SSOP (derate 7.00mW/°C above +70°C) .........559mW
20-Pin TSSOP (derate 8.0mW/°C above +70°C).............640mW
28-Pin TSSOP (derate 8.7mW/°C above +70°C).............696mW
28-Pin SSOP (derate 9.52mW/°C above +70°C) .........762mW
28-Pin SO (derate 12.50mW/°C above +70°C) .....................1W
Operating Temperature Ranges
MAX32_ _C_ _.....................................................0°C to +70°C
MAX32_ _E_ _ .................................................-40°C to +85°C
Storage Temperature Range .............................-65°C to +150°C
Lead Temperature (soldering, 10s) .................................+300°C
IOUT = -1.0mA
IOUT = 1.6mA
Receivers disabled
T_IN, EN, SHDN, MBAUD
T_IN, EN, SHDN, MBAUD
CONDITIONS
V
0.8 1.5
Input Threshold Low
0.6 1.2
Input Voltage Range -25 25 V
0.5 2.0
VCC Power-Supply Current
Output Voltage High VCC - 0.6 VCC - 0.1 V
Output Voltage Low 0.4 V
Output Leakage Current ±0.05 ±10 μA
Input Leakage Current ±0.01 ±1.0 μA
0.8 V
Input Logic Threshold Low
(Note 3)
PARAMETER MIN TYP MAX UNITS
TA = +25°C
TA = +25°C V
1.8 2.4
Input Threshold High
1.5 2.4
VCC = 3.3V
VCC = 5.0V
2.0
V
2.4
Input Logic Threshold High
(Note 3)
No load, VCC = 3.3V or 5.0V,
TA = +25°C
mA
0.3 1.0
MAX3222/MAX3232/
MAX3241
MAX3237
Shutdown Supply Current SHDN = GND, TA = +25°C 1.0 10 μA
VCC = 3.3V
VCC = 5.0V
VCC = 3.3V
VCC = 5.0V
DC CHARACTERISTICS
LOGIC INPUTS AND RECEIVER OUTPUTS
RECEIVER INPUTS
MAX3222/MAX3232/MAX3237/MAX3241
3.0V to 5.5V, Low-Power, up to 1Mbps, True RS-232
Transceivers Using Four 0.1μF External Capacitors
_______________________________________________________________________________________ 3
TIMING CHARACTERISTICS—MAX3222/MAX3232/MAX3241
(VCC = +3.0V to +5.5V, C1–C4 = 0.1μF (Note 2), TA = TMIN to TMAX, unless otherwise noted. Typical values are at TA = +25°C.)
ELECTRICAL CHARACTERISTICS (continued)
(VCC = +3.0V to +5.5V, C1–C4 = 0.1μF (Note 2), TA = TMIN to TMAX, unless otherwise noted. Typical values are at TA = +25°C.)
T1IN = T2IN = GND, T3IN = VCC,
T3OUT loaded with 3kΩ to GND,
T1OUT and T2OUT loaded with 2.5mA each
CONDITIONS
Transmitter Output Voltage ±5.0 V
Input Hysteresis 0.3 V
PARAMETER MIN TYP MAX UNITS
Output Voltage Swing All transmitter outputs loaded with 3kΩ to ground ±5.0 ±5.4 V
Output Short-Circuit Current ±35 ±60 mA
Output Resistance VCC = V+ = V- = 0V, VOUT = ±2V 300 10M Ω
VOUT = ±12V, VCC = 0V or 3V to 5.5V, transmitters
disabled
Output Leakage Current ±25 μA
tPHL
RL = 3kΩ, CL = 1000pF, one transmitter switching
tPLH
| tPHL - tPLH |
| tPHL - tPLH |
Normal operation
Normal operation
CONDITIONS
0.3
μs
0.3
Receiver Propagation Delay
Maximum Data Rate 120 235 kbps
Receiver Skew 300 ns
Transmitter Skew 300 ns
Receiver Output Disable Time 200 ns
Receiver Output Enable Time 200 ns
PARAMETER MIN TYP MAX UNITS
VCC = 3.3V, RL = 3kΩ to 7kΩ, 6 30
+3V to -3V or -3V to +3V,
TA = +25°C, one transmitter
switching
V/μs
4 30
Transition-Region Slew Rate
R_IN to R_OUT, CL = 150pF
CL = 150pF to
1000pF
CL = 150pF to
2500pF
Input Resistance TA = +25°C 3 5 7 kΩ
MOUSE DRIVEABILITY (MAX3241)
TRANSMITTER OUTPUTS
MAX3222/MAX3232/MAX3237/MAX3241
3.0V to 5.5V, Low-Power, up to 1Mbps, True RS-232
Transceivers Using Four 0.1μF External Capacitors
4 _______________________________________________________________________________________
__________________________________________Typical Operating Characteristics
(VCC = +3.3V, 235kbps data rate, 0.1μF capacitors, all transmitters loaded with 3kΩ, TA = +25°C, unless otherwise noted.)
RL = 3kΩ, CL = 1000pF, one transmitter switching,
MBAUD = GND
Normal operation
CONDITIONS
Receiver Output Disable Time Normal operation 200 ns
| tPHL - tPLH |, MBAUD = GND 100 ns
0.15
250
μs
0.15
Receiver Propagation Delay
Receiver Output Enable Time 200 ns
PARAMETER MIN TYP MAX UNITS
TIMING CHARACTERISTICS—MAX3237
(VCC = +3.0V to +5.5V, C1–C4 = 0.1μF (Note 2), TA = TMIN to TMAX, unless otherwise noted. Typical values are at TA = +25°C.)
VCC = 3.0V to 4.5V, RL = 3kΩ, CL = 250pF,
one transmitter switching, MBAUD = VCC
1000
VCC = 4.5V to 5.5V, RL = 3kΩ, CL = 1000pF,
one transmitter switching, MBAUD = VCC
kbps
1000
Maximum Data Rate
R_IN to R_OUT, CL = 150pF
| tPHL - tPLH |, MBAUD = VCC 25 ns
Transmitter Skew
Receiver Skew | tPHL - tPLH | 50 ns
6 30
V/μs
4 30
tPHL
tPLH
CL = 150pF to 2500pF,
MBAUD = GND
CL = 150pF
to 1000pF
MBAUD =
GND
VCC = 3.3V, RL = 3Ω to 7kΩ,
+3V to -3V or -3V to +3V,
TA = +25°C
Transition-Region Slew Rate MBAUD =
VCC
24 150
Note 2: MAX3222/MAX3232/MAX3241: C1–C4 = 0.1μF tested at 3.3V ±10%; C1 = 0.047μF, C2–C4 = 0.33μF tested at 5.0V ±10%.
MAX3237: C1–C4 = 0.1μF tested at 3.3V ±5%; C1–C4 = 0.22μF tested at 3.3V ±10%; C1 = 0.047μF, C2–C4 = 0.33μF tested
at 5.0V ±10%.
Note 3: Transmitter input hysteresis is typically 250mV.
-6
-5
-4
-3
-2
-1
0
1
2
3
4
5
6
0
MAX3222/MAX3232
TRANSMITTER OUTPUT VOLTAGE
vs. LOAD CAPACITANCE
MAX3222-01
LOAD CAPACITANCE (pF)
TRANSMITTER OUTPUT VOLTAGE (V)
1000 2000 3000 4000 5000
VOUT+
VOUT-
0
2
4
6
8
10
12
14
16
18
20
22
150
MAX3222/MAX3232
SLEW RATE
vs. LOAD CAPACITANCE
MAX3222-02
LOAD CAPACITANCE (pF)
SLEW RATE (V/μs)
1000 2000 3000 4000 5000
FOR DATA RATES UP TO 235kbps
+SLEW
-SLEW
0
5
10
15
20
25
30
35
40
0
MAX3222/MAX3232
SUPPLY CURRENT vs. LOAD CAPACITANCE
WHEN TRANSMITTING DATA
MAX3222-03
LOAD CAPACITANCE (pF)
SUPPLY CURRENT (mA)
1000 2000 3000 4000 5000
235kbps
120kbps
20kbps
MAX3222/MAX3232/MAX3237/MAX3241
3.0V to 5.5V, Low-Power, up to 1Mbps, True RS-232
Transceivers Using Four 0.1μF External Capacitors
_______________________________________________________________________________________ 5
-7.5
-5.0
-2.5
0
2.5
5.0
7.5
0
MAX3241
TRANSMITTER OUTPUT VOLTAGE
vs. LOAD CAPACITANCE
MAX3222-04
LOAD CAPACITANCE (pF)
TRANSMITTER OUTPUT VOLTAGE (V)
1000 2000 3000 4000 5000
VOUT+
1 TRANSMITTER AT 235kbps
2 TRANSMITTERS AT 30kbps
VOUTALL
OUTPUTS LOADED WITH 3kΩ +CL
0.1μF CHARGE-PUMP CAPACITORS
4 FOR ALL DATA RATES UP TO 235kbps
6
8
10
12
14
16
18
20
22
24
0
MAX3241
SLEW RATE
vs. LOAD CAPACITANCE
MAX3222-05
LOAD CAPACITANCE (pF)
SLEW RATE (V/μs)
1000 2000 3000 4000 5000
+SLEW
-SLEW
0
5
10
15
20
25
30
35
45
40
0
MAX3241
SUPPLY CURRENT vs. LOAD
CAPACITANCE WHEN TRANSMITTING DATA
MAX3222-06
LOAD CAPACITANCE (pF)
SUPPLY CURRENT (mA)
1000 2000 3000 4000 5000
235kbps
120kbps
20kbps
-7.5
-5.0
-2.5
0
2.5
5.0
7.5
0
MAX3237
TRANSMITTER OUTPUT VOLTAGE
vs. LOAD CAPACITANCE (MBAUD = GND)
MAX3222-07
LOAD CAPACITANCE (pF)
TRANSMITTER OUTPUT VOLTAGE (V)
1000 2000 3000 4000 5000
1 TRANSMITTER AT 240kbps
4 TRANSMITTERS AT 15kbps
3kΩ + CL LOADS
VCC = 3.3V
0
10
20
30
50
40
60
70
0
MAX3237
SLEW RATE vs. LOAD CAPACITANCE
(MBAUD = VCC)
MAX3222-10
LOAD CAPACITANCE (pF)
SLEW RATE (V/μs)
500 1000 1500 2000
-SLEW, 1Mbps
+SLEW, 1Mbps
1 TRANSMITTER AT FULL DATA RATE
4 TRANSMITTERS AT 1/16 DATA RATE
3kΩ + CL LOAD EACH OUTPUT
VCC = 3.3V
-SLEW, 2Mbps
+SLEW, 2Mbps
-7.5
-5.0
-2.5
0
2.5
5.0
7.5
0
MAX3237
TRANSMITTER OUTPUT VOLTAGE
vs. LOAD CAPACITANCE (MBAUD = VCC)
MAX3222-08
LOAD CAPACITANCE (pF)
TRANSMITTER OUTPUT VOLTAGE (V)
500 1000 1500 2000
1 TRANSMITTER AT FULL DATA RATE
4 TRANSMITTERS AT 1/16 DATA RATE
3kΩ + CL LOAD, EACH OUTPUT
VCC = 3.3V
2Mbps 1.5Mbps
1Mbps
2Mbps
1Mbps
1.5Mbps
0
10
20
30
40
50
60
0
MAX3237
SUPPLY CURRENT vs.
LOAD CAPACITANCE (MBAUD = GND)
MAX3222-11
LOAD CAPACITANCE (pF)
SUPPLY CURRENT (mA)
1000 2000 3000 4000 5000
240kbps
120kbps
20kbps
1 TRANSMITTER AT FULL DATA RATE
4 TRANSMITTERS AT 1/16 DATA RATE
3kΩ + CL LOADS
VCC = 3.3V
0
2
4
6
8
10
12
0
MAX3237
SLEW RATE vs. LOAD CAPACITANCE
(MBAUD = GND)
MAX3222-09
LOAD CAPACITANCE (pF)
SLEW RATE (V/μs)
1000 2000 3000 4000 5000
+SLEW
-SLEW
1 TRANSMITTER AT 240kbps
4 TRANSMITTERS AT 15kbps
3kΩ + CL LOADS
VCC = 3.3V
0
10
30
20
40
50
60
70
0
MAX3237
SKEW vs. LOAD CAPACITANCE
(tPLH - tPHL)
MAX3222-12
LOAD CAPACITANCE (pF)
500 1000 1500 2000 2500
MAX
MIN
AVERAGE; 10 PARTS
SKEW (ns)
1 TRANSMITTER AT 512kbps
4 TRANSMITTERS AT 32kbps
3kΩ + CL LOADS
VCC = 3.3V
MBAUD = VCC
_____________________________Typical Operating Characteristics (continued)
(VCC = +3.3V, 235kbps data rate, 0.1μF capacitors, all transmitters loaded with 3kΩ, TA = +25°C, unless otherwise noted.)
MAX3222/MAX3232/MAX3237/MAX3241
3.0V to 5.5V, Low-Power, up to 1Mbps, True RS-232
Transceivers Using Four 0.1μF External Capacitors
6 _______________________________________________________________________________________
—
FUNCTION
—
MAX3222
Noninverting Complementary Receiver Outputs.
Always active.
DIP/SO SSOP
— 11, 14
1 1 Receiver Enable. Active low.
2 2
Positive Terminal of Voltage-Doubler Charge-Pump
Capacitor
6 6
Negative Terminal of Inverting Charge-Pump
Capacitor
5 5
Positive Terminal of Inverting Charge-Pump
Capacitor
4 4
Negative Terminal of Voltage-Doubler Charge-Pump
Capacitor
3 3 +5.5V Generated by the Charge Pump
11, 12 12, 13 TTL/CMOS Transmitter Inputs
10, 13 10, 15 TTL/CMOS Receiver Outputs
9, 14 9, 16 RS-232 Receiver Inputs
8, 15 8, 17 RS-232 Transmitter Outputs
7 7 -5.5V Generated by the Charge Pump
18 20 Shutdown Control. Active low.
17 19 +3.0V to +5.5V Supply Voltage
16 18 Ground
No Connection
MAX3232 MAX3237
— 16
— 13
1 28
5 3
4 1
3 25
2 27
10, 11
17, 19, 22,
23, 24
9, 12 18, 20, 21
8, 13 8, 9, 11
7, 14
5, 6, 7,
10, 12
6 4
— 14
16 26
15 2
— —
NAME
EN
C1+
C2-
C2+
C1-
V+
T_IN
R_OUT
R_IN
T_OUT
VSHDN
VCC
GND
R_OUTB
N.C.
MAX3241
20, 21
23
28
2
1
24
27
12, 13, 14
15–19
4–8
9, 10, 11
3
22
26
25
—
PIN
— —
MegaBaud Control Input. Connect to GND for normal
operation; connect to VCC for 1Mbps transmission rates.
— 15 — MBAUD
______________________________________________________________Pin Description
MAX3222/MAX3232/MAX3237/MAX3241
_______________Detailed Description
Dual Charge-Pump Voltage Converter
The MAX3222/MAX3232/MAX3237/MAX3241’s internal
power supply consists of a regulated dual charge pump
that provides output voltages of +5.5V (doubling charge
pump) and -5.5V (inverting charge pump), regardless of
the input voltage (VCC) over the 3.0V to 5.5V range. The
charge pumps operate in a discontinuous mode; if the
output voltages are less than 5.5V, the charge pumps
are enabled, and if the output voltages exceed 5.5V, the
charge pumps are disabled. Each charge pump
requires a flying capacitor (C1, C2) and a reservoir
capacitor (C3, C4) to generate the V+ and V- supplies.
RS-232 Transmitters
The transmitters are inverting level translators that convert
CMOS-logic levels to 5.0V EIA/TIA-232 levels.
The MAX3222/MAX3232/MAX3241 transmitters guarantee
a 120kbps data rate with worst-case loads of 3kΩ in
parallel with 1000pF, providing compatibility with PC-to-
PC communication software (such as LapLink™).
Typically, these three devices can operate at data rates
of 235kbps. Transmitters can be paralleled to drive multiple
receivers or mice.
The MAX3222/MAX3237/MAX3241’s output stage is
turned off (high impedance) when the device is in shutdown
mode. When the power is off, the MAX3222/
MAX3232/MAX3237/MAX3241 permit the outputs to be
driven up to ±12V.
The transmitter inputs do not have pullup resistors.
Connect unused inputs to GND or VCC.
MAX3237 MegaBaud Operation
In normal operating mode (MBAUD = GND), the
MAX3237 transmitters guarantee a 250kbps data rate
with worst-case loads of 3kΩ in parallel with 1000pF.
This provides compatibility with PC-to-PC communication
software, such as LapLink.
For higher speed serial communications, the MAX3237
features MegaBaud operation. In MegaBaud operating
mode (MBAUD = VCC), the MAX3237 transmitters guarantee
a 1Mbps data rate with worst-case loads of 3kΩ in
parallel with 250pF for 3.0V < VCC < 4.5V. For 5V ±10%
operation, the MAX3237 transmitters guarantee a 1Mbps
data rate into worst-case loads of 3kΩ in parallel with
1000pF.
MAX3222
MAX3232
MAX3237
MAX3241
5kΩ
R_ OUT R_ IN
EN*
C2-
C2+
C1-
C1+
VV+
VCC
C4
C1 C3
C2
0.1μF
VCC
SHDN*
T_ IN T_ OUT
GND
VCC
0V
7kΩ 150pF
MAX3222
MAX3232
MAX3237
MAX3241
5kΩ
R_ OUT R_ IN
EN*
C2-
C2+
C1-
C1+
VV+
VCC
C4
C1 C3
C2
0.1μF
VCC
SHDN*
T_ IN T_ OUT
GND
VCC
0V
3kΩ 2500pF
MINIMUM SLEW-RATE TEST CIRCUIT MAXIMUM SLEW-RATE TEST CIRCUIT
*MAX3222/MAX3237/MAX3241 ONLY
Figure 1. Slew-Rate Test Circuits
LapLink is a trademark of Traveling Software, Inc.
3.0V to 5.5V, Low-Power, up to 1Mbps, True RS-232
Transceivers Using Four 0.1μF External Capacitors
_______________________________________________________________________________________ 7
MAX3222/MAX3232/MAX3237/MAX3241
3.0V to 5.5V, Low-Power, up to 1Mbps, True RS-232
Transceivers Using Four 0.1μF External Capacitors
8 _______________________________________________________________________________________
RS-232 Receivers
The receivers convert RS-232 signals to CMOS-logic output
levels. The MAX3222/MAX3237/MAX3241 receivers
have inverting three-state outputs. In shutdown, the
receivers can be active or inactive (Table 1).
The complementary outputs on the MAX3237 (R1OUTB)
and the MAX3241 (R1OUTB, R2OUTB) are always active,
regardless of the state of EN or SHDN. This allows for
Ring Indicator applications without forward biasing other
devices connected to the receiver outputs. This is ideal
for systems where VCC is set to 0V in shutdown to
accommodate peripherals, such as UARTs (Figure 2).
MAX3222/MAX3237/MAX3241
Shutdown Mode
Supply current falls to less than 1μA in shutdown mode
(SHDN = low). When shut down, the device’s charge
pumps are turned off, V+ is pulled down to VCC, V- is
pulled to ground, and the transmitter outputs are disabled
(high impedance). The time required to exit shutdown
is typically 100μs, as shown in Figure 3. Connect
SHDN to VCC if the shutdown mode is not used. SHDN
has no effect on R_OUT or R_OUTB.
MAX3222/MAX3237/MAX3241
Enable Control
The inverting receiver outputs (R_OUT) are put into a
high-impedance state when EN is high. The complementary
outputs R1OUTB and R2OUTB are always active,
regardless of the state of EN and SHDN (Table 1). EN
has no effect on T_OUT.
__________Applications Information
Capacitor Selection
The capacitor type used for C1–C4 is not critical for
proper operation; polarized or nonpolarized capacitors
can be used. The charge pump requires 0.1μF capacitors
for 3.3V operation. For other supply voltages, refer to
Table 2 for required capacitor values. Do not use values
lower than those listed in Table 2. Increasing the capacitor
values (e.g., by a factor of 2) reduces ripple on the
transmitter outputs and slightly reduces power consumption.
C2, C3, and C4 can be increased without changing
C1’s value. However, do not increase C1 without also
increasing the values of C2, C3, and C4, to maintain the
proper ratios (C1 to the other capacitors).
When using the minimum required capacitor values,
make sure the capacitor value does not degrade excessively
with temperature. If in doubt, use capacitors with a
higher nominal value. The capacitor’s equivalent series
resistance (ESR), which usually rises at low temperatures,
influences the amount of ripple on V+ and V-.
MAX3237
MAX3241
T1OUT
R1OUTB
Tx
UART
VCC
T1IN
LOGIC
TRANSITION
DETECTOR
R1OUT R1IN
EN = VCC
SHDN = GND
VCC
TO
μP
Rx
PREVIOUS
RS-232
Tx
UART
PROTECTION
DIODE
PROTECTION
DIODE
SHDN = GND
VCC
VCC
GND
Rx
5kΩ
a) OLDER RS-232: POWERED-DOWN UART DRAWS CURRENT FROM
ACTIVE RECEIVER OUTPUT IN SHUTDOWN.
b) NEW MAX3237/MAX3241: EN SHUTS DOWN RECEIVER OUTPUTS (EXCEPT FOR
B OUTPUTS), SO NO CURRENT FLOWS TO UART IN SHUTDOWN. B OUTPUTS
INDICATE RECEIVER ACTIVITY DURING SHUTDOWN WITH EN HIGH.
GND
5kΩ
Figure 2. Detection of RS-232 Activity when the UART and
Interface are Shut Down; Comparison of MAX3237/MAX3241
(b) with Previous Transceivers (a).
MAX3222/MAX3232/MAX3237/MAX3241
3.0V to 5.5V, Low-Power, up to 1Mbps, True RS-232
Transceivers Using Four 0.1μF External Capacitors
_______________________________________________________________________________________ 9
Power-Supply Decoupling
In most circumstances, a 0.1μF bypass capacitor is
adequate. In applications that are sensitive to powersupply
noise, decouple VCC to ground with a capacitor
of the same value as charge-pump capacitor C1. Connect
bypass capacitors as close to the IC as possible.
Operation Down to 2.7V
Transmitter outputs will meet EIA/TIA-562 levels of
±3.7V with supply voltages as low as 2.7V.
Transmitter Outputs when
Exiting Shutdown
Figure 3 shows two transmitter outputs when exiting
shutdown mode. As they become active, the two transmitter
outputs are shown going to opposite RS-232 levels
(one transmitter input is high, the other is low).
Each transmitter is loaded with 3kΩ in parallel with
2500pF. The transmitter outputs display no ringing or
undesirable transients as they come out of shutdown.
Note that the transmitters are enabled only when the
magnitude of V- exceeds approximately 3V.
Mouse Driveability
The MAX3241 has been specifically designed to power
serial mice while operating from low-voltage power supplies.
It has been tested with leading mouse brands from
manufacturers such as Microsoft and Logitech. The
MAX3241 successfully drove all serial mice tested and
met their respective current and voltage requirements.
Figure 4a shows the transmitter output voltages under
increasing load current at 3.0V. Figure 4b shows a typical
mouse connection using the MAX3241.
Table 1. MAX3222/MAX3237/MAX3241
Shutdown and Enable Control Truth Table
Table 2. Required Minimum Capacitor Values
5V/div
VCC = 3.3V
C1–C4 = 0.1μF
2V/div
T2
50μs/div
T1
Figure 3. Transmitter Outputs when Exiting Shutdown or
Powering Up
VCC
(V)
C1
(μF)
4.5 to 5.5 0.047
3.0 to 5.5 0.1
C2, C3, C4
(μF)
MAX3222/MAX3232/MAX3241
0.33
0.47
1 Active
0
1
1 Active
SHDN
0 1 High-Z
0 0 High-Z
EN T_OUT
High-Z
Active
High-Z
Active
R_OUT
R_OUTB
(MAX3237/
MAX3241)
Active
Active
Active
Active
3.0 to 3.6 0.22
3.15 to 3.6 0.1
MAX3237
0.22
0.1
4.5 to 5.5 0.047
3.0 to 5.5 0.22
0.33
1.0
3.0 to 3.6 0.1 0.1
MAX3222/MAX3232/MAX3237/MAX3241
3.0V to 5.5V, Low-Power, up to 1Mbps, True RS-232
Transceivers Using Four 0.1μF External Capacitors
10 ______________________________________________________________________________________
MAX3241
23 EN
15 R5OUT
16 R4OUT
17 R3OUT
18 R2OUT
19 R1OUT
20 R2OUTB
21 R1OUTB
5kΩ
5kΩ
5kΩ
5kΩ
5kΩ
R5IN 8
R4IN 7
6
R2IN 5
R1IN 4
SHDN 22
GND
25
12 T3IN
13 T2IN
14 T1IN
2 C2-
1 C2+
24 C1-
28 C1+
T3OUT 11
+V
COMPUTER SERIAL PORT
MOUSE
+V
-V
GND
Tx
T2OUT 10
T1OUT 9
V-
3
V+
VCC 27
VCC
C4
C1 C3
C2
0.1μF
VCC = 3V
to 5.5V
26
R3IN
Figure 4b. Mouse Driver Test Circuit
-6
-5
-4
-3
-2
-1
0
1
2
3
4
5
6
0 1 2 3 4 5 6 7
MAX3222-04
LOAD CURRENT PER TRANSMITTER (mA)
TRANSMITTER OUTPUT VOLTAGE (V)
VOUT+
VCC = 3.0V
VOUTVOUT+
VCC VOUTT1
T2
T3
Figure 4a. MAX3241 Transmitter Output Voltage vs. Load Current per Transmitter
MAX3222/MAX3232/MAX3237/MAX3241
3.0V to 5.5V, Low-Power, up to 1Mbps, True RS-232
Transceivers Using Four 0.1μF External Capacitors
______________________________________________________________________________________ 11
High Data Rates
The MAX3222/MAX3232/MAX3241 maintain the RS-232
±5.0V minimum transmitter output voltage even at high
data rates. Figure 5 shows a transmitter loopback test
circuit. Figure 6 shows a loopback test result at
120kbps, and Figure 7 shows the same test at 235kbps.
For Figure 6, all transmitters were driven simultaneously
at 120kbps into RS-232 loads in parallel with 1000pF.
For Figure 7, a single transmitter was driven at 235kbps,
and all transmitters were loaded with an RS-232 receiver
in parallel with 1000pF.
The MAX3237 maintains the RS-232 ±5.0V minimum
transmitter output voltage at data rates up to 1Mbps.
Figure 8 shows a loopback test result at 1Mbps with
MBAUD = VCC. For Figure 8, all transmitters were
loaded with an RS-232 receiver in parallel with 250pF.
MAX3222
MAX3232
MAX3237
MAX3241
5kΩ
R_ OUT R_ IN
EN*
C2-
C2+
C1-
C1+
VV+
VCC
C4
C1 C3
C2
0.1μF
VCC
SHDN*
T_ IN T_ OUT
GND
VCC
0V 1000pF
*MAX3222/MAX3237/MAX3241 ONLY
T1IN
R1OUT
VCC = 3.3V
T1OUT
5V/div
5V/div
5V/div
5μs/div
Figure 5. Loopback Test Circuit
Figure 6. MAX3241 Loopback Test Result at 120kbps
T1IN
R1OUT
VCC = 3.3V
T1OUT
5V/div
5V/div
2μs/div
5V/div
Figure 7. MAX3241 Loopback Test Result at 235kbps
+5V
0V
+5V
0V
-5V
+5V
0V
T_IN
T_OUT = R_IN
5kΩ + 250pF
R_OUT
150pF
200ns/div
VCC = 3.3V
Figure 8. MAX3237 Loopback Test Result at 1000kbps
(MBAUD = VCC)
MAX3222/MAX3232/MAX3237/MAX3241
3.0V to 5.5V, Low-Power, up to 1Mbps, True RS-232
Transceivers Using Four 0.1μF External Capacitors
12 ______________________________________________________________________________________
__________________________________________________Typical Operating Circuits
MAX3222
10 R2OUT
1
13 R1OUT
R2IN 9
18
GND
16
RS-232
OUTPUTS
TTL/CMOS
INPUTS
11 T2IN
12 T1IN
C2-
6
5 C2+
4 C1-
2 C1+
R1IN 14
T2OUT 8
T1OUT 15
V-
7
V+
VCC 3
17
C1
0.1μF
C2
0.1μF
0.1μF
+3.3V
RS-232
INPUTS
TTL/CMOS
OUTPUTS
EN
SHDN
C3*
0.1μF
C4
0.1μF
PIN NUMBERS REFER TO DIP/SO PACKAGES.
* C3 CAN BE RETURNED TO EITHER VCC OR GROUND.
MAX3232
9 R2OUT
12 R1OUT
R2IN 8
GND
15
RS-232
OUTPUTS
TTL/CMOS
INPUTS
10 T2IN
11 T1IN
C2-
5
4 C2+
3 C1-
1 C1+
R1IN 13
T2OUT 7
T1OUT 14
V-
6
V+
VCC 2
C4
0.1μF
16
0.1μF
0.1μF
0.1μF
+3.3V
RS-232
INPUTS
TTL/CMOS
OUTPUTS
C3*
0.1μF
* C3 CAN BE RETURNED TO EITHER VCC OR GROUND.
SEE TABLE 2 FOR CAPACITOR SELECTION
5kΩ
5kΩ
5kΩ
5kΩ
Interconnection with 3V and 5V Logic
The MAX3222/MAX3232/MAX3237/MAX3241 can
directly interface with various 5V logic families, including
ACT and HCT CMOS. See Table 3 for more information
on possible combinations of interconnections.
Table 3. Logic-Family Compatibility
with Various Supply Voltages
Compatible with ACT and
HCT CMOS, and with TTL.
Incompatible with AC, HC,
and CD4000 CMOS.
5 3.3
SYSTEM
POWERSUPPLY
VOLTAGE
(V)
Compatible with all TTL
and CMOS-logic families.
5 5
Compatible with all CMOS
families.
3.3 3.3
COMPATIBILITY
MAX32_ _
VCC
SUPPLY
VOLTAGE
(V)
MAX3222/MAX3232/MAX3237/MAX3241
_____________________________________Typical Operating Circuits (continued)
MAX3241
23 EN
15 R5OUT
16 R4OUT
17 R3OUT
18 R2OUT
19 R1OUT
20 R2OUTB
21 R1OUTB
TTL/CMOS
OUTPUTS
5kΩ
5kΩ
5kΩ
5kΩ
5kΩ
R5IN 8
* C3 CAN BE RETURNED TO EITHER VCC OR GROUND.
R4IN 7
R3IN 6
R2IN 5
R1IN 4
RS-232
INPUTS
SHDN 22
GND
25
RS-232
OUTPUTS
TTL/CMOS
INPUTS
12 T3IN
13 T2IN
14 T1IN
C2-
2
1 C2+
24 C1-
28 C1+
T3OUT 11
T2OUT 10
T1OUT 9
V-
3
V+
VCC 27
C4
0.1μF
C3*
0.1μF
0.1μF
0.1μF
0.1μF
+3.3V
26
MAX3237
13 EN
18 R3OUT
20 R2OUT
21 R1OUT
16 R1OUTB
LOGIC
OUTPUTS
5kΩ
5kΩ
5kΩ
* C3 CAN BE RETURNED TO EITHER VCC OR GROUND.
R3IN 11
R2IN 9
R1IN 8
RS-232
INPUTS
GND
2
RS-232
OUTPUTS
LOGIC
INPUTS
22 T3IN
23 T2IN
24 T1IN
C2-
3
1 C2+
25 C1-
28 C1+
T3OUT 7
T2OUT 6
T1OUT 5
T1
T2
T3
R1
R2
R3
V-
4
V+
VCC 27
0.1μF
0.1μF
0.1μF
0.1μF
0.1μF
26
MBAUD 15
17 T5IN
19 T4IN
T5OUT 12
T4OUT 10
SHDN
14
T4
T5
3.0V to 5.5V, Low-Power, up to 1Mbps, True RS-232
Transceivers Using Four 0.1μF External Capacitors
______________________________________________________________________________________ 13
MAX3222/MAX3232/MAX3237/MAX3241
3.0V to 5.5V, Low-Power, up to 1Mbps, True RS-232
Transceivers Using Four 0.1μF External Capacitors
14 ______________________________________________________________________________________
_____________________________________________Pin Configurations (continued)
16
15
14
13
12
11
10
9
1
2
3
4
5
6
7
8
VCC
GND
T1OUT
C2+ R1IN
C1-
V+
C1+
MAX3232
R1OUT
T1IN
T2IN
R2IN R2OUT
T2OUT
VC2-
DIP/SO/SSOP/TSSOP
+
TOP VIEW
20
19
18
17
16
15
14
13
1
2
3
8
12
10 11
4
5
6
7
SHDN
VCC
GND
C1- T1OUT
V+
C1+
EN
R1IN
R1OUT
T1IN
T2IN
T2OUT
VC2-
C2+
R2IN 9
R2OUT
SSOP/TSSOP
+
N.C.
N.C.
MAX3222
28
27
26
25
24
23
22
21
20
19
18
17
16
15
1
2
3
4
5
6
7
8
9
10
11
12
13
14
C1+
V+
VCC
C1-
T1IN
T2IN
MBAUD
T3IN
R1OUT
R2OUT
T4IN
R3OUT
T5IN
R1OUTB
SHDN
EN
T5OUT
R3IN
T4OUT
R2IN
R1IN
T3OUT
T2OUT
T1OUT
VC2-
GND
C2+
SSOP
MAX3237
+
28
27
26
25
24
23
22
21
20
19
18
17
16
15
1
2
3
4
5
6
7
8
9
10
11
12
13
14
C1+
V+
VCC
GND
C1-
EN
R5OUT
SHDN
R1OUTB
R2OUTB
R1OUT
R2OUT
R3OUT
R4OUT
T1IN
T2IN
T3IN
T3OUT
T2OUT
T1OUT
R5IN
R4IN
R3IN
R2IN
R1IN
VC2-
C2+
SO/SSOP/TSSOP
MAX3241
+
MAX3222/MAX3232/MAX3237/MAX3241
3.0V to 5.5V, Low-Power, up to 1Mbps, True RS-232
Transceivers Using Four 0.1μF External Capacitors
______________________________________________________________________________________ 15
______3V-Powered EIA/TIA-232 and EIA/TIA-562 Transceivers from Maxim
Ordering Information (continued)
*Dice are tested at TA = +25°C, DC parameters only.
+Denotes lead-free package.
0.1μF capacitors, 1 complementary
receiver, MegaBaud
operation
MAX3237 3.0 to 5.5 5/3 3 250/1000 232
0.1μF capacitors, AutoShutdown,
complementary receiver,
drives mice
MAX3243 3.0 to 5.5 3/5 1 120 232
MAX3232 3.0 to 5.5 2/2 N/A 120 232 0.1μF capacitors
MAX3223 3.0 to 5.5 2/2 2 120 232 0.1μF capacitors, AutoShutdown
MAX3222 3.0 to 5.5 2/2 2 120 232 0.1μF capacitors
232
562
232
562
232
EIA/
TIA-232
OR 562
2.7 to 3.6
AutoShutdown, complementary
receiver, drives mice, transient
detection
MAX3212 3/5 5 235
MAX563 3.0 to 3.6 2/2 2 230 0.1μF capacitors
Operates directly from batteries
without a voltage regulator
MAX218 1.8 to 4.25 2/2 2 120
No. OF
RECEIVERS
ACTIVE IN
SHUTDOWN
POWERSUPPLY
VOLTAGE
(V)
MAX562 2.7 to 5.25 3/5 5 230 Wide supply range
MAX212 3.0 to 3.6 3/5 5 120 Drives mice
FEATURES
GUARANTEED
DATA
RATE
(kbps)
No. OF
TRANSMITTERS/
RECEIVERS
PART
0.1μF capacitors, 2 complementary
receivers, drives mice
MAX3241 3.0 to 5.5 3/5 5 120 232
PART TEMP RANGE PIN-PACKAGE
PKG
CODE
MAX3222EUP+ -40°C to +85°C 20 TSSOP U20+2
MAX3222EAP+ -40°C to +85°C 20 SSOP A20+1
MAX3222EWN+ -40°C to +85°C 18 SO W18+1
MAX3222EPN+ -40°C to +85°C 18 Plastic Dip P18+5
MAX3222C/D 0°C to +70°C Dice* —
MAX3232CUE+ 0°C to +70°C 16 TSSOP U16+1
MAX3232CSE+ 0°C to +70°C 16 Narrow SO S16+1
MAX3232CWE+ 0°C to +70°C 16 Wide SO W16+1
MAX3232CPE+ 0°C to +70°C 16 Plastic DIP P16+1
MAX3232EUE+ -40°C to +85°C 16 TSSOP U16+1
MAX3232ESE+ -40°C to +85°C 16 Narrow SO S16+5
PART TEMP RANGE PIN-PACKAGE
PKG
CODE
MAX3232EWE+ -40°C to +85°C 16 Wide SO W16+1
MAX3232EPE+ -40°C to +85°C 16 Plastic DIP P16+1
MAX3232CAE+ 0°C to +70°C 16 SSOP A28+2
MAX3237CAI+ 0°C to +70°C 28 SSOP A28+1
MAX3237ENI+ -40°C to +85°C 28 SSOP A28+1
MAX3241CAI+ 0°C to +70°C 28 SSOP A28+1
MAX3241CWI+ 0°C to +70°C 28 SO W28+6
MAX3241EUI+ -40°C to +85°C 28 TSSOP U28+2
MAX3241EAI+ -40°C to +85°C 28 SSOP A28+1
MAX3241EWI+ -40°C to +85°C 28 SO W28+6
MAX3222/MAX3232/MAX3237/MAX3241
3.0V to 5.5V, Low-Power, up to 1Mbps, True RS-232
Transceivers Using Four 0.1μF External Capacitors
16 ______________________________________________________________________________________
___________________Chip Topography ___________________Chip Information
T2IN T1IN
0.127"
(3.225mm)
0.087"
(2.209mm)
T2OUT R2IN R2OUT
R1OUT
R1IN
T1OUT
V+ C1+ VCC
SHDN
EN
C1-
C2+
C2-
VGND
MAX3222
TRANSISTOR COUNT: 339
SUBSTRATE CONNECTED TO GND
MAX3222 339
MAX3232 339
MAX3237 1212
MAX3241 894
PART TRANSISTOR COUNT
3.0V to 5.5V, Low-Power, up to 1Mbps, True RS-232
Transceivers Using Four 0.1μF External Capacitors
Maxim cannot assume responsibility for use of any circuitry other than circuitry entirely embodied in a Maxim product. No circuit patent licenses are
implied. Maxim reserves the right to change the circuitry and specifications without notice at any time.
Maxim Integrated Products, 120 San Gabriel Drive, Sunnyvale, CA 94086 408-737-7600 ____________________ 17
© 2007 Maxim Integrated Products Maxim is a registered trademark of Maxim Integrated Products, Inc.
MAX3222/MAX3232/MAX3237/MAX3241
TSSOP4.40mm.EPS
Package Information
(The package drawing(s) in this data sheet may not reflect the most current specifications. For the latest package outline information,
go to www.maxim-ic.com/packages.)
Revision History
Pages changed at Rev 7: 1, 15, 16, 17
Precision Micropower,
Low Dropout Voltage References
Data Sheet REF19x Series
Rev. L
Information furnished by Analog Devices is believed to be accurate and reliable. However, no
responsibility is assumed by Analog Devices for its use, nor for any infringements of patents or other
rights of third parties that may result from its use. Specifications subject to change without notice. No
license is granted by implication or otherwise under any patent or patent rights of Analog Devices.
Trademarks and registered trademarks are the property of their respective owners.
One Technology Way, P.O. Box 9106, Norwood, MA 02062-9106, U.S.A.
Tel: 781.329.4700 www.analog.com
Fax: 781.461.3113 ©1996–2011 Analog Devices, Inc. All rights reserved.
FEATURES
Temperature coefficient: 5 ppm/°C maximum
High output current: 30 mA
Low supply current: 45 μA maximum
Initial accuracy: ±2 mV maximum1
Sleep mode: 15 μA maximum
Low dropout voltage
Load regulation: 4 ppm/mA
Line regulation: 4 ppm/V
Short-circuit protection
APPLICATIONS
Portable instruments
ADCs and DACs
Smart sensors
Solar powered applications
Loop-current-powered instruments
GENERAL DESCRIPTION
The REF19x series precision band gap voltage references use a patented temperature drift curvature correction circuit and laser trimming of highly stable, thin-film resistors to achieve a very low temperature coefficient and high initial accuracy.
The REF19x series is made up of micropower, low dropout voltage (LDV) devices, providing stable output voltage from supplies as low as 100 mV above the output voltage and consuming less than 45 μA of supply current. In sleep mode, which is enabled by applying a low TTL or CMOS level to the SLEEP pin, the output is turned off and supply current is further reduced to less than 15 μA.
The REF19x series references are specified over the extended industrial temperature range (−40°C to +85°C) with typical performance specifications over −40°C to +125°C for applications, such as automotive.
All electrical grades are available in an 8-lead SOIC package; the PDIP and TSSOP packages are available only in the lowest electrical grade.
TEST PINS
Test Pin 1 and Test Pin 5 are reserved for in-package Zener zap. To achieve the highest level of accuracy at the output, the Zener zapping technique is used to trim the output voltage. Because each unit may require a different amount of adjustment, the resistance value at the test pins varies widely from pin to pin and from part to part. The user should leave Pin 1 and Pin 5 unconnected. REF19xSERIESTOP VIEW(Not to Scale)TP1VS2SLEEP3GND4NCNCOUTPUTTP876500371-001NOTES1.NC = NO CONNECT.2.TP PINS ARE FACTORY TESTPOINTS, NO USER CONNECTION.
Figure 1. 8-Lead SOIC_N and TSSOP Pin Configuration (S Suffix and RU Suffix) REF19xSERIESTOP VIEW(Not to Scale)TP1VS2SLEEP3GND4NCNCOUTPUTTP876500371-002NOTES1.NC = NO CONNECT.2.TP PINS ARE FACTORY TESTPOINTS, NO USER CONNECTION.
Figure 2. 8-Lead PDIP Pin Configuration (P Suffix)
Table 1. Nominal Output Voltage
Part Number
Nominal Output Voltage (V)
REF191
2.048
REF192
2.50
REF193
3.00
REF194
4.50
REF195
5.00
REF196
3.30
REF198
4.096
1 Initial accuracy does not include shift due to solder heat effect (see the Applications Information section).
FEATURES
l VERY LOW NOISE: 4.5nV/ÖHz at 10kHz
l FAST SETTLING TIME:
OPA627—550ns to 0.01%
OPA637—450ns to 0.01%
l LOW VOS: 100mV max
l LOW DRIFT: 0.8mV/°C max
l LOW IB: 5pA max
l OPA627: Unity-Gain Stable
l OPA637: Stable in Gain ³ 5
OPA627
OPA637
DESCRIPTION
The OPA627 and OPA637 Difet operational amplifiers
provide a new level of performance in a precision
FET op amp. When compared to the popular OPA111
op amp, the OPA627/637 has lower noise, lower offset
voltage, and much higher speed. It is useful in a broad
range of precision and high speed analog circuitry.
The OPA627/637 is fabricated on a high-speed, dielectrically-
isolated complementary NPN/PNP process. It
operates over a wide range of power supply voltage—
±4.5V to ±18V. Laser-trimmed Difet input circuitry
provides high accuracy and low-noise performance
comparable with the best bipolar-input op amps.
High frequency complementary transistors allow increased
circuit bandwidth, attaining dynamic performance
not possible with previous precision FET op
amps. The OPA627 is unity-gain stable. The OPA637
is stable in gains equal to or greater than five.
Difet fabrication achieves extremely low input bias
currents without compromising input voltage noise
performance. Low input bias current is maintained
over a wide input common-mode voltage range with
unique cascode circuitry.
The OPA627/637 is available in plastic DIP, SOIC
and metal TO-99 packages. Industrial and military
temperature range models are available.
Difet ®, Burr-Brown Corp.
®
Precision High-Speed
Difet ® OPERATIONAL AMPLIFIERS
APPLICATIONS
l PRECISION INSTRUMENTATION
l FAST DATA ACQUISITION
l DAC OUTPUT AMPLIFIER
l OPTOELECTRONICS
l SONAR, ULTRASOUND
l HIGH-IMPEDANCE SENSOR AMPS
l HIGH-PERFORMANCE AUDIO CIRCUITRY
l ACTIVE FILTERS
Trim
5
Trim
1
+In
3
–In
2
Output
6
7
+VS
–VS
4
©1989 Burr-Brown Corporation PDS-998H Printed in U.S.A. March, 1998
International Airport Industrial Park • Mailing Address: PO Box 11400, Tucson, AZ 85734 • Street Address: 6730 S. Tucson Blvd., Tucson, AZ 85706 • Tel: (520) 746-1111 • Twx: 910-952-1111
Internet: http://www.burr-brown.com/ • FAXLine: (800) 548-6133 (US/Canada Only) • Cable: BBRCORP • Telex: 066-6491 • FAX: (520) 889-1510 • Immediate Product Info: (800) 548-6132
OPA627
OPA627
SBOS165
2
®
OPA627, 637
SPECIFICATIONS
ELECTRICAL
At TA = +25°C, and VS = ±15V, unless otherwise noted.
OPA627BM, BP, SM OPA627AM, AP, AU
OPA637BM, BP, SM OPA637AM, AP, AU
PARAMETER CONDITIONS MIN TYP MAX MIN TYP MAX UNITS
OFFSET VOLTAGE (1)
Input Offset Voltage 40 100 130 250 mV
AP, BP, AU Grades 100 250 280 500 mV
Average Drift 0.4 0.8 1.2 2 mV/°C
AP, BP, AU Grades 0.8 2 2.5 mV/°C
Power Supply Rejection VS = ±4.5 to ±18V 106 120 100 116 dB
INPUT BIAS CURRENT (2)
Input Bias Current VCM = 0V 1 5 2 10 pA
Over Specified Temperature VCM = 0V 1 2 nA
SM Grade VCM = 0V 50 nA
Over Common-Mode Voltage VCM = ±10V 1 2 pA
Input Offset Current VCM = 0V 0.5 5 1 10 pA
Over Specified Temperature VCM = 0V 1 2 nA
SM Grade 50 nA
NOISE
Input Voltage Noise
Noise Density: f = 10Hz 15 40 20 nV/ÖHz
f = 100Hz 8 20 10 nV/ÖHz
f = 1kHz 5.2 8 5.6 nV/ÖHz
f = 10kHz 4.5 6 4.8 nV/ÖHz
Voltage Noise, BW = 0.1Hz to 10Hz 0.6 1.6 0.8 mVp-p
Input Bias Current Noise
Noise Density, f = 100Hz 1.6 2.5 2.5 fA/ÖHz
Current Noise, BW = 0.1Hz to 10Hz 30 60 48 fAp-p
INPUT IMPEDANCE
Differential 1013 || 8 * W || pF
Common-Mode 1013 || 7 * W || pF
INPUT VOLTAGE RANGE
Common-Mode Input Range ±11 ±11.5 * * V
Over Specified Temperature ±10.5 ±11 * * V
Common-Mode Rejection VCM = ±10.5V 106 116 100 110 dB
OPEN-LOOP GAIN
Open-Loop Voltage Gain VO = ±10V, RL = 1kW 112 120 106 116 dB
Over Specified Temperature VO = ±10V, RL = 1kW 106 117 100 110 dB
SM Grade VO = ±10V, RL = 1kW 100 114 dB
FREQUENCY RESPONSE
Slew Rate: OPA627 G = –1, 10V Step 40 55 * * V/ms
OPA637 G = –4, 10V Step 100 135 * * V/ms
Settling Time: OPA627 0.01% G = –1, 10V Step 550 * ns
0.1% G = –1, 10V Step 450 * ns
OPA637 0.01% G = –4, 10V Step 450 * ns
0.1% G = –4, 10V Step 300 * ns
Gain-Bandwidth Product: OPA627 G = 1 16 * MHz
OPA637 G = 10 80 * MHz
Total Harmonic Distortion + Noise G = +1, f = 1kHz 0.00003 * %
POWER SUPPLY
Specified Operating Voltage ±15 * V
Operating Voltage Range ±4.5 ±18 * * V
Current ±7 ±7.5 * * mA
OUTPUT
Voltage Output RL = 1kW ±11.5 ±12.3 * *
Over Specified Temperature ±11 ±11.5 * * V
Current Output VO = ±10V ±45 * mA
Short-Circuit Current ±35 +70/–55 ±100 * * * mA
Output Impedance, Open-Loop 1MHz 55 * W
TEMPERATURE RANGE
Specification: AP, BP, AM, BM, AU –25 +85 * * °C
SM –55 +125 °C
Storage: AM, BM, SM –60 +150 * * °C
AP, BP, AU –40 +125 * * °C
qJ-A: AM, BM, SM 200 * °C/W
AP, BP 100 * °C/W
AU 160 °C/W
* Specifications same as “B” grade.
NOTES: (1) Offset voltage measured fully warmed-up. (2) High-speed test at TJ = +25°C. See Typical Performance Curves for warmed-up performance.
The information provided herein is believed to be reliable; however, BURR-BROWN assumes no responsibility for inaccuracies or omissions. BURR-BROWN assumes
no responsibility for the use of this information, and all use of such information shall be entirely at the user’s own risk. Prices and specifications are subject to change
without notice. No patent rights or licenses to any of the circuits described herein are implied or granted to any third party. BURR-BROWN does not authorize or warrant
any BURR-BROWN product for use in life support devices and/or systems.
3
®
OPA627, 637
PIN CONFIGURATIONS
Top View DIP/SOIC
Offset Trim
–In
+In
–V
No Internal Connection
+V
Output
S Offset Trim
S
1
2
3
4
8
7
6
5
Top View TO-99
Offset Trim
–In Output
+In Offset Trim
–VS
+VS
No Internal Connection
Case connected to –VS.
8
1
2
3
4
5
6
7
ABSOLUTE MAXIMUM RATINGS(1)
Supply Voltage .................................................................................. ±18V
Input Voltage Range .............................................. +VS + 2V to –VS – 2V
Differential Input Range ....................................................... Total VS + 4V
Power Dissipation ........................................................................ 1000mW
Operating Temperature
M Package .................................................................. –55°C to +125°C
P, U Package ............................................................. –40°C to +125°C
Storage Temperature
M Package .................................................................. –65°C to +150°C
P, U Package ............................................................. –40°C to +125°C
Junction Temperature
M Package .................................................................................. +175°C
P, U Package ............................................................................. +150°C
Lead Temperature (soldering, 10s) ............................................... +300°C
SOlC (soldering, 3s) ................................................................... +260°C
NOTE: (1) Stresses above these ratings may cause permanent damage.
ELECTROSTATIC
DISCHARGE SENSITIVITY
This integrated circuit can be damaged by ESD. Burr-Brown
recommends that all integrated circuits be handled with
appropriate precautions. Failure to observe proper handling
and installation procedures can cause damage.
ESD damage can range from subtle performance degradation
to complete device failure. Precision integrated circuits
may be more susceptible to damage because very small
parametric changes could cause the device not to meet its
published specifications.
PACKAGE/ORDERING INFORMATION
PACKAGE DRAWING TEMPERATURE
PRODUCT PACKAGE NUMBER(1) RANGE
OPA627AP Plastic DIP 006 –25°C to +85°C
OPA627BP Plastic DIP 006 –25°C to +85°C
OPA627AU SOIC 182 –25°C to +85°C
OPA627AM TO-99 Metal 001 –25°C to +85°C
OPA627BM TO-99 Metal 001 –25°C to +85°C
OPA627SM TO-99 Metal 001 –55°C to +125°C
OPA637AP Plastic DIP 006 –25°C to +85°C
OPA637BP Plastic DIP 006 –25°C to +85°C
OPA637AU SOIC 182 –25°C to +85°C
OPA637AM TO-99 Metal 001 –25°C to +85°C
OPA637BM TO-99 Metal 001 –25°C to +85°C
OPA637SM TO-99 Metal 001 –55°C to +125°C
NOTE: (1) For detailed drawing and dimension table, please see end of data
sheet, or Appendix C of Burr-Brown IC Data Book.
4
®
OPA627, 637
TYPICAL PERFORMANCE CURVES
At TA = +25°C, and VS = ±15V, unless otherwise noted.
INPUT VOLTAGE NOISE SPECTRAL DENSITY
1k
100
10
1
1
Frequency (Hz)
Voltage Noise (nV/ Ö Hz)
10 100 1k 10k 100k 1M 10M
VOLTAGE NOISE vs SOURCE RESISTANCE
Source Resistance ( W )
1k
100
10
1
100
OPA627 + Resistor
Resistor Noise Only
Spot Noise
at 10kHz
Voltage Noise (nV/ Ö Hz)
1k 10k 100k 1M 10M 100M
Comparison with
OPA27 Bipolar Op
Amp + Resistor
–
+
RS
OPA627 GAIN/PHASE vs FREQUENCY
Phase (Degrees)
Gain (dB)
30
20
10
0
–10
–90
–120
–150
–180
–210
1
Phase
Gain
Frequency (MHz)
10 100
75° Phase
Margin
OPA637 GAIN/PHASE vs FREQUENCY
Phase (Degrees)
Gain (dB)
30
20
10
0
–10
–90
–120
–150
–180
–210
1 10 100
Phase
Gain
Frequency (MHz)
TOTAL INPUT VOLTAGE NOISE vs BANDWIDTH
100
10
1
0.1
0.01
1 10 100 1k 10k 100k 1M 10M
Bandwidth (Hz)
Input Voltage Noise (μV)
Noise Bandwidth:
0.1Hz to indicated
frequency.
RMS
p-p
OPEN-LOOP GAIN vs FREQUENCY
Frequency (Hz)
Voltage Gain (dB)
1 10 100 1k 10k 100k 1M 10M 100M
140
120
100
80
60
40
20
0
–20
OPA637
OPA627
5
®
OPA627, 637
TYPICAL PERFORMANCE CURVES (CONT)
At TA = +25°C, and VS = ±15V, unless otherwise noted.
OPEN-LOOP GAIN vs TEMPERATURE
Voltage Gain (dB)
Temperature (°C)
125
120
115
110
105
–75 –50 –25 0 25 50 75 100 125
OPEN-LOOP OUTPUT IMPEDANCE vs FREQUENCY
Frequency (Hz)
Output Resistance (W)
100
80
60
40
20
0
2 20 200 2k 20k 200k 2M 20M
COMMON-MODE REJECTION vs FREQUENCY
Frequency (Hz)
Common-Mode Rejection Ratio (dB)
140
120
100
80
60
40
20
0
1 10 100 1k 10k 100k 1M 10M
OPA627
OPA637
COMMON-MODE REJECTION vs
INPUT COMMON MODE VOLTAGE
130
120
110
100
90
80
Common-Mode Rejection (dB)
Common-Mode Voltage (V)
–15 –10 –5 0 5 10 15
POWER-SUPPLY REJECTION vs FREQUENCY
Frequency (Hz)
Power-Supply Rejection (dB)
140
120
100
80
60
40
20
0
1
–VS PSRR 627
and 637
+VS PSRR 627
637
10 100 1k 10k 100k 1M 10M
POWER-SUPPLY REJECTION AND COMMON-MODE
REJECTION vs TEMPERATURE
Temperature (°C)
CMR and PSR (dB)
125
120
115
110
105
–75
PSR
CMR
–50 –25 0 25 50 75 100 125
6
®
OPA627, 637
TYPICAL PERFORMANCE CURVES (CONT)
At TA = +25°C, and VS = ±15V, unless otherwise noted.
SUPPLY CURRENT vs TEMPERATURE
Temperature (°C)
Supply Current (mA)
8
7.5
7
6.5
6
–75 –50 –25 0 25 50 75 100 125
OUTPUT CURRENT LIMIT vs TEMPERATURE
Output Current (mA)
100
80
60
40
20
0
–75 –50 –25 0 25 50 75 100 125
Temperature (°C)
–IL at VO = –10V
–IL at VO = 0V
+IL at VO = +10V
+IL at VO = 0V
OPA627 GAIN-BANDWIDTH AND SLEW RATE
vs TEMPERATURE
Temperature (°C)
Gain-Bandwidth (MHz)
24
20
16
12
8
–75
Slew Rate
GBW
60
55
50
Slew Rate (V/μs)
–50 –25 0 25 50 75 100 125
OPA637 GAIN-BANDWIDTH AND SLEW RATE
vs TEMPERATURE
Temperature (°C)
Gain-Bandwidth (MHz)
120
100
80
60
40
–75
Slew Rate (V/μs)
160
140
120
100
80
Slew Rate
GBW
–50 –25 0 25 50 75 100 125
OPA627 TOTAL HARMONIC DISTORTION + NOISE
vs FREQUENCY
Frequency (Hz)
THD+N (%)
20 100 1k 10k 20k
0.1
0.01
0.001
0.0001
0.00001
G = +10
G = +1
Measurement BW: 80kHz
–
+
–
+
100pF 100pF
G = +1 G = +10
VI VI
549
5k
600 600
VO = ±10V VO = ±10V
W W
W
W
OPA637 TOTAL HARMONIC DISTORTION + NOISE
vs FREQUENCY
Frequency (Hz)
THD+N (%)
20 100 1k 10k 20k
1
0.1
0.01
0.001
0.0001
G = +10
G = +50
–
+
100pF
G = +10
VI
549
5k
600
VO = ±10V
W
W
W
–
+
100pF
G = +50
VI
102
5k
600
VO = ±10V
W
W
W
Measurement BW: 80kHz
7
®
OPA627, 637
TYPICAL PERFORMANCE CURVES (CONT)
At TA = +25°C, and VS = ±15V, unless otherwise noted.
INPUT BIAS AND OFFSET CURRENT
vs JUNCTION TEMPERATURE
Junction Temperature (°C)
Input Current (pA)
10k
1k
100
10
1
0.1
–50 –25 0 25 50 75 100 125 150
IB
IOS
INPUT BIAS CURRENT
vs POWER SUPPLY VOLTAGE
Supply Voltage (±VS)
Input Bias Current (pA)
20
15
10
5
0
±4 ±6 ±8 ±10 ±12 ±14 ±16 ±18
NOTE: Measured fully
warmed-up.
TO-99 with 0807HS Heat Sink
TO-99
Plastic
DIP, SOIC
INPUT BIAS CURRENT vs COMMON-MODE VOLTAGE
Common-Mode Voltage (V)
Input Bias Current Multiplier
1.2
1.1
1
0.9
0.8
–15 –10 –5 0 5 10 15
Beyond Linear
Common-Mode Range
Beyond Linear
Common-Mode Range
INPUT OFFSET VOLTAGE WARM-UP vs TIME
Time From Power Turn-On (Min)
Offset Voltage Change (μV)
50
25
0
–25
–50
0 1 2 3 4 5 6
MAX OUTPUT VOLTAGE vs FREQUENCY
Frequency (Hz)
Output Voltage (Vp-p)
30
20
10
0
100k 1M 10M 100M
OPA627
OPA637
SETTLING TIME vs CLOSED-LOOP GAIN
100
10
1
0.1
–1 –10 –100 –1000
Closed-Loop Gain (V/V)
Settling Time (μs)
Error Band: ±0.01%
OPA637
OPA627
8
®
OPA627, 637
TYPICAL PERFORMANCE CURVES (CONT)
At TA = +25°C, and VS = ±15V, unless otherwise noted.
FIGURE 1. Circuits with Noise Gain Less than Five Require
the OPA627 for Proper Stability.
SETTLING TIME vs ERROR BAND
1500
1000
500
0
0.001 0.01 0.1 1 10
Error Band (%)
Settling Time (ns)
OPA637
G = –4
OPA627
G = –1
–
+
CF
RI RF
2kW
+5V
–5V
OPA627 OPA637
RI 2kW 500W
RF 2kW 2kW
CF 6pF 4pF
SETTLING TIME vs LOAD CAPACITANCE
0 150 200 300 400 500
Load Capacitance (pF)
3
2
1
0
Settling Time (μs)
Error Band:
±0.01%
OPA637
G = –4
OPA627
G = –1
APPLICATIONS INFORMATION
The OPA627 is unity-gain stable. The OPA637 may be used
to achieve higher speed and bandwidth in circuits with noise
gain greater than five. Noise gain refers to the closed-loop
gain of a circuit as if the non-inverting op amp input were
being driven. For example, the OPA637 may be used in a
non-inverting amplifier with gain greater than five, or an
inverting amplifier of gain greater than four.
When choosing between the OPA627 or OPA637, it is
important to consider the high frequency noise gain of your
circuit configuration. Circuits with a feedback capacitor
(Figure 1) place the op amp in unity noise-gain at high
frequency. These applications must use the OPA627 for
proper stability. An exception is the circuit in Figure 2,
where a small feedback capacitance is used to compensate
for the input capacitance at the op amp’s inverting input. In
this case, the closed-loop noise gain remains constant with
frequency, so if the closed-loop gain is equal to five or
greater, the OPA637 may be used.
–
+
–
+
–
+
–
+
–
+
–
+
Buffer
Bandwidth
Limiting
Integrator Filter
RI
RF < 4R
Inverting Amp
G < |–4|
RI
RF < 4RI
Non-Inverting Amp
G < 5
OPA627 OPA627
OPA627 OPA627
OPA627 OPA627
9
®
OPA627, 637
–
+
C2
C1 R2
R1
OPA637
C1 = CIN + CSTRAY
C2 =
R1 C1
R2
OFFSET VOLTAGE ADJUSTMENT
The OPA627/637 is laser-trimmed for low offset voltage
and drift, so many circuits will not require external adjustment.
Figure 3 shows the optional connection of an external
potentiometer to adjust offset voltage. This adjustment should
not be used to compensate for offsets created elsewhere in a
system (such as in later amplification stages or in an A/D
converter) because this could introduce excessive temperature
drift. Generally, the offset drift will change by approximately
4mV/°C for 1mV of change in the offset voltage due
to an offset adjustment (as shown on Figure 3).
FIGURE 2. Circuits with Noise Gain Equal to or Greater than
Five May Use the OPA637.
amp contributes little additional noise. Below 1kW, op amp
noise dominates over the resistor noise, but compares
favorably with precision bipolar op amps.
CIRCUIT LAYOUT
As with any high speed, wide bandwidth circuit, careful
layout will ensure best performance. Make short, direct
interconnections and avoid stray wiring capacitance—especially
at the input pins and feedback circuitry.
The case (TO-99 metal package only) is internally connected
to the negative power supply as it is with most common op
amps. Pin 8 of the plastic DIP, SOIC, and TO-99 packages
has no internal connection.
Power supply connections should be bypassed with good
high frequency capacitors positioned close to the op amp
pins. In most cases 0.1mF ceramic capacitors are adequate.
The OPA627/637 is capable of high output current (in
excess of 45mA). Applications with low impedance loads or
capacitive loads with fast transient signals demand large
currents from the power supplies. Larger bypass capacitors
such as 1mF solid tantalum capacitors may improve dynamic
performance in these applications.
NOISE PERFORMANCE
Some bipolar op amps may provide lower voltage noise
performance, but both voltage noise and bias current noise
contribute to the total noise of a system. The OPA627/637
is unique in providing very low voltage noise and very low
current noise. This provides optimum noise performance
over a wide range of sources, including reactive source
impedances. This can be seen in the performance curve
showing the noise of a source resistor combined with the
noise of an OPA627. Above a 2kW source resistance, the op
FIGURE 4. Connection of Input Guard for Lowest IB.
Board Layout for Input Guarding:
Guard top and bottom of board.
Alternate—use Teflon® standoff for sensitive
input pins.
Teflon® E.I. du Pont de Nemours & Co.
–
+
2
In 3
Non-inverting
6
OPA627
Out
–
+
2
3
In
Inverting
6
OPA627
Out
–
+
2
In 3
Buffer
6
OPA627
Out
3
2
4
5
6
7
8 No Internal Connection
1
TO-99 Bottom View
To Guard Drive
–
+
2
3
7
1
5
6
+VS
–VS
OPA627/637
100kW
10kW to 1MW
Potentiometer
(100kW preferred)
±10mV Typical
Trim Range
4
FIGURE 3. Optional Offset Voltage Trim Circuit.
10
®
OPA627, 637
takes approximately 500ns. When the output is driven into
the positive limit, recovery takes approximately 6ms. Output
recovery of the OPA627 can be improved using the output
clamp circuit shown in Figure 5. Diodes at the inverting
input prevent degradation of input bias current.
INPUT BIAS CURRENT
Difet fabrication of the OPA627/637 provides very low
input bias current. Since the gate current of a FET doubles
approximately every 10°C, to achieve lowest input bias
current, the die temperature should be kept as low as possible.
The high speed and therefore higher quiescent current
of the OPA627/637 can lead to higher chip temperature. A
simple press-on heat sink such as the Burr-Brown model
807HS (TO-99 metal package) can reduce chip temperature
by approximately 15°C, lowering the IB to one-third its
warmed-up value. The 807HS heat sink can also reduce lowfrequency
voltage noise caused by air currents and thermoelectric
effects. See the data sheet on the 807HS for details.
Temperature rise in the plastic DIP and SOIC packages can
be minimized by soldering the device to the circuit board.
Wide copper traces will also help dissipate heat.
The OPA627/637 may also be operated at reduced power
supply voltage to minimize power dissipation and temperature
rise. Using ±5V power supplies reduces power dissipation
to one-third of that at ±15V. This reduces the IB of TO-
99 metal package devices to approximately one-fourth the
value at ±15V.
Leakage currents between printed circuit board traces can
easily exceed the input bias current of the OPA627/637. A
circuit board “guard” pattern (Figure 4) reduces leakage
effects. By surrounding critical high impedance input circuitry
with a low impedance circuit connection at the same
potential, leakage current will flow harmlessly to the lowimpedance
node. The case (TO-99 metal package only) is
internally connected to –VS.
Input bias current may also be degraded by improper handling
or cleaning. Contamination from handling parts and
circuit boards may be removed with cleaning solvents and
deionized water. Each rinsing operation should be followed
by a 30-minute bake at 85°C.
Many FET-input op amps exhibit large changes in input
bias current with changes in input voltage. Input stage
cascode circuitry makes the input bias current of the
OPA627/637 virtually constant with wide common-mode
voltage changes. This is ideal for accurate high inputimpedance
buffer applications.
PHASE-REVERSAL PROTECTION
The OPA627/637 has internal phase-reversal protection.
Many FET-input op amps exhibit a phase reversal when the
input is driven beyond its linear common-mode range. This
is most often encountered in non-inverting circuits when the
input is driven below –12V, causing the output to reverse
into the positive rail. The input circuitry of the OPA627/637
does not induce phase reversal with excessive commonmode
voltage, so the output limits into the appropriate rail.
OUTPUT OVERLOAD
When the inputs to the OPA627/637 are overdriven, the
output voltage of the OPA627/637 smoothly limits at approximately
2.5V from the positive and negative power
supplies. If driven to the negative swing limit, recovery
+VS
5kW
(2)
HP 5082-2811
1kW
5kW
–VS
VO
Diode Bridge
BB: PWS740-3
ZD1 : 10V IN961
Clamps output
at VO = ±11.5V
RI
VI –
+
RF
ZD1
OPA627
FIGURE 5. Clamp Circuit for Improved Overload Recovery.
CAPACITIVE LOADS
As with any high-speed op amp, best dynamic performance
can be achieved by minimizing the capacitive load. Since a
load capacitance presents a decreasing impedance at higher
frequency, a load capacitance which is easily driven by a
slow op amp can cause a high-speed op amp to perform
poorly. See the typical curves showing settling times as a
function of capacitive load. The lower bandwidth of the
OPA627 makes it the better choice for driving large capacitive
loads. Figure 6 shows a circuit for driving very large
load capacitance. This circuit’s two-pole response can also
be used to sharply limit system bandwidth. This is often
useful in reducing the noise of systems which do not require
the full bandwidth of the OPA627.
FIGURE 6. Driving Large Capacitive Loads.
R1
–
+
RF
1kW
OPA627
CF
G = +1
BW 1MHz
200pF
For Approximate Butterworth Response:
CF =
2 RO CL
RF
RF >> RO
G = 1+
RF
R1
³
Optional Gain
Gain > 1
f–3dB =
1
2p Ö RF RO CF CL
CL
5nF
RO
20W
11
®
OPA627, 637
INPUT PROTECTION
The inputs of the OPA627/637 are protected for voltages
between +VS + 2V and –VS – 2V. If the input voltage can
exceed these limits, the amplifier should be protected. The
diode clamps shown in Figure 7a will prevent the input
voltage from exceeding one forward diode voltage drop
beyond the power supplies—well within the safe limits. If
the input source can deliver current in excess of the maximum
forward current of the protection diodes, use a series
resistor, RS, to limit the current. Be aware that adding
resistance to the input will increase noise. The 4nV/ÖHz
theoretical thermal noise of a 1kW resistor will add to the
4.5nV/ÖHz noise of the OPA627/637 (by the square-root of
the sum of the squares), producing a total noise of 6nV/ÖHz.
Resistors below 100W add negligible noise.
Leakage current in the protection diodes can increase the
total input bias current of the circuit. The specified maximum
leakage current for commonly used diodes such as the
1N4148 is approximately 25nA—more than a thousand
times larger than the input bias current of the OPA627/637.
Leakage current of these diodes is typically much lower and
may be adequate in many applications. Light falling on the
junction of the protection diodes can dramatically increase
leakage current, so common glass-packaged diodes should
be shielded from ambient light. Very low leakage can be
achieved by using a diode-connected FET as shown. The
2N4117A is specified at 1pA and its metal case shields the
junction from light.
Sometimes input protection is required on I/V converters of
inverting amplifiers (Figure 7b). Although in normal operation,
the voltage at the summing junction will be near zero
(equal to the offset voltage of the amplifier), large input
transients may cause this node to exceed 2V beyond the
power supplies. In this case, the summing junction should
be protected with diode clamps connected to ground. Even
with the low voltage present at the summing junction,
common signal diodes may have excessive leakage current.
Since the reverse voltage on these diodes is clamped, a
diode-connected signal transistor can be used as an inexpensive
low leakage diode (Figure 7b).
FIGURE 7. Input Protection Circuits.
–
+
–VS
+VS
Optional RS
VO
D: IN4148 — 25nA Leakage
2N4117A — 1pA Leakage
(a)
=
–
+
IIN
VO
D
D
D
(b)
D
D: 2N3904
=
NC
Siliconix
OPA627
OPA627
FPO
When used as a unity-gain buffer, large common-mode input voltage steps
produce transient variations in input-stage currents. This causes the rising
edge to be slower and falling edges to be faster than nominal slew rates
observed in higher-gain circuits.
(A) (B)
LARGE SIGNAL RESPONSE SMALL SIGNAL RESPONSE
FIGURE 8. OPA627 Dynamic Performance, G = +1.
–
+
OPA627
G = 1
12
®
OPA627, 637
When driven with a very fast input step (left), common-mode
transients cause a slight variation in input stage currents which
will reduce output slew rate. If the input step slew rate is reduced
(right), output slew rate will increase slightly.
FIGURE 9. OPA627 Dynamic Performance, G = –1.
NOTE: (1) Optimum value will
depend on circuit board layout
and stray capacitance at
the inverting input.
LARGE SIGNAL RESPONSE
+10
0
–10
VOUT (V)
+10
0
–10
(C) (D)
OPA637
LARGE SIGNAL RESPONSE
OPA637
SMALL SIGNAL RESPONSE
FPO
FIGURE 10. OPA637 Dynamic Response, G = 5.
–10
0
+10
–100
0
+100
(E) (F)
VOUT (V)
–
+
OPA627
G = –1
2kW
2kW
6pF(1)
VOUT
–
+
OPA637
G = 5
2kW
500W
4pF(1)
VOUT
NOTE: (1) Optimum value will depend on circuit
board layout and capacitance at inverting input.
VOUT (V)
VOUT (mV)
13
®
OPA627, 637
OPA627 OPA637
RI, R1 2kW 500W
CF 6pF 4pF
Error Band ±0.5mV ±0.2mV
(0.01%)
NOTE: CF is selected for best settling time performance
depending on test fixture layout. Once optimum value is
determined, a fixed capacitor may be used.
FIGURE 12. High Speed Instrumentation Amplifier, Gain = 100.
–In
+In
+
–
OPA637
Differential Voltage Gain = 1 + 2RF/RG
2
3
–
+
–
+
INA105
Differential
Amplifier
1
6
5
Output
Gain = 100
CMRR 116dB
Bandwidth 1MHz
OPA637
25kW
25kW
25kW
25kW
Input Common-Mode
Range = ±5V
»
»
3pF
RF
5kW
RF
5kW
RG
101W
–
+
±5V
Out
+15V
2kW
CF
2kW
Error Out
RI
RI
51W
–15V
HP-
5082-
2835
High Quality
Pulse Generator
/
FIGURE 11. Settling Time and Slew Rate Test Circuit.
FIGURE 14. Composite Amplifier for Wide Bandwidth.
This composite amplifier uses the OPA603 current-feedback op amp to
provide extended bandwidth and slew rate at high closed-loop gain. The
feedback loop is closed around the composite amp, preserving the
precision input characteristics of the OPA627/637. Use separate power
supply bypass capacitors for each op amp.
GAIN A1 R1 R2 R3 R4 –3dB SLEW RATE
(V/V) OP AMP (W) (kW) (W) (kW) (MHz) (V/ms)
100 OPA627 50.5(1) 4.99 20 1 15 700
1000 OPA637 49.9 4.99 12 1 11 500
NOTE: (1) Closest 1/2% value.
*Minimize capacitance at this node.
FIGURE 13. High Speed Instrumentation Amplifier, Gain = 1000.
+
–
OPA603
–
+
A1
R3
R1
R4
R2
VI VO
*
RL ³ 150W
for ±10V Out
–In
+In
+
–
OPA637
Differential Voltage Gain = (1 + 2RF/RG) • 10
2
3
–
+
–
+
INA106
Differential
Amplifier
1
6
5
Output
Gain = 1000
CMRR 116dB
Bandwidth 400kHz
OPA637
10kW
10kW
100kW
100kW
Input Common-Mode
Range = ±10V
»
»
3pF
RF
5kW
RF
5kW
RG
101W
PACKAGE OPTION ADDENDUM
www.ti.com 10-Jun-2014
Addendum-Page 1
PACKAGING INFORMATION
Orderable Device Status
(1)
Package Type Package
Drawing
Pins Package
Qty
Eco Plan
(2)
Lead/Ball Finish
(6)
MSL Peak Temp
(3)
Op Temp (°C) Device Marking
(4/5)
Samples
OPA627AM NRND TO-99 LMC 8 20 Green (RoHS
& no Sb/Br)
AU N / A for Pkg Type OPA627AM
OPA627AP ACTIVE PDIP P 8 50 TBD Call TI Call TI OPA627AP
OPA627APG4 ACTIVE PDIP P 8 50 TBD Call TI Call TI OPA627AP
OPA627AU ACTIVE SOIC D 8 75 Green (RoHS
& no Sb/Br)
CU NIPDAU Level-3-260C-168 HR -25 to 85 OPA
627AU
OPA627AU/2K5 ACTIVE SOIC D 8 2500 Green (RoHS
& no Sb/Br)
CU NIPDAU Level-3-260C-168 HR -25 to 85 OPA
627AU
OPA627AU/2K5E4 ACTIVE SOIC D 8 2500 Green (RoHS
& no Sb/Br)
CU NIPDAU Level-3-260C-168 HR -25 to 85 OPA
627AU
OPA627AUE4 ACTIVE SOIC D 8 75 Green (RoHS
& no Sb/Br)
CU NIPDAU Level-3-260C-168 HR -25 to 85 OPA
627AU
OPA627AUG4 ACTIVE SOIC D 8 75 Green (RoHS
& no Sb/Br)
CU NIPDAU Level-3-260C-168 HR -25 to 85 OPA
627AU
OPA627BM NRND TO-99 LMC 8 1 Green (RoHS
& no Sb/Br)
AU N / A for Pkg Type OPA627BM
OPA627BP ACTIVE PDIP P 8 50 TBD Call TI Call TI OPA627BP
OPA627BPG4 ACTIVE PDIP P 8 50 TBD Call TI Call TI OPA627BP
OPA627SM NRND TO-99 LMC 8 20 Green (RoHS
& no Sb/Br)
AU N / A for Pkg Type OPA627SM
OPA637AM NRND TO-99 LMC 8 20 Green (RoHS
& no Sb/Br)
AU N / A for Pkg Type OPA637AM
OPA637AM2 OBSOLETE TO-99 LMC 8 TBD Call TI Call TI
OPA637AP ACTIVE PDIP P 8 50 TBD Call TI Call TI OPA637AP
OPA637APG4 ACTIVE PDIP P 8 50 TBD Call TI Call TI OPA637AP
OPA637AU ACTIVE SOIC D 8 75 Green (RoHS
& no Sb/Br)
CU NIPDAU Level-3-260C-168 HR -25 to 85 OPA
637AU
OPA637AU/2K5 ACTIVE SOIC D 8 2500 Green (RoHS
& no Sb/Br)
CU NIPDAU Level-3-260C-168 HR -25 to 85 OPA
637AU
OPA637AUE4 OBSOLETE SOIC D 8 TBD Call TI Call TI -25 to 85
PACKAGE OPTION ADDENDUM
www.ti.com 10-Jun-2014
Addendum-Page 2
Orderable Device Status
(1)
Package Type Package
Drawing
Pins Package
Qty
Eco Plan
(2)
Lead/Ball Finish
(6)
MSL Peak Temp
(3)
Op Temp (°C) Device Marking
(4/5)
Samples
OPA637AUG4 ACTIVE SOIC D 8 75 Green (RoHS
& no Sb/Br)
CU NIPDAU Level-3-260C-168 HR -25 to 85 OPA
637AU
OPA637BM NRND TO-99 LMC 8 20 Green (RoHS
& no Sb/Br)
AU N / A for Pkg Type OPA637BM
OPA637BM1 OBSOLETE TO-99 LMC 8 TBD Call TI Call TI
OPA637BP ACTIVE PDIP P 8 50 TBD Call TI Call TI OPA637BP
OPA637BPG4 ACTIVE PDIP P 8 50 TBD Call TI Call TI OPA637BP
OPA637SM NRND TO-99 LMC 8 20 Green (RoHS
& no Sb/Br)
AU N / A for Pkg Type OPA637SM
(1) The marketing status values are defined as follows:
ACTIVE: Product device recommended for new designs.
LIFEBUY: TI has announced that the device will be discontinued, and a lifetime-buy period is in effect.
NRND: Not recommended for new designs. Device is in production to support existing customers, but TI does not recommend using this part in a new design.
PREVIEW: Device has been announced but is not in production. Samples may or may not be available.
OBSOLETE: TI has discontinued the production of the device.
(2) Eco Plan - The planned eco-friendly classification: Pb-Free (RoHS), Pb-Free (RoHS Exempt), or Green (RoHS & no Sb/Br) - please check http://www.ti.com/productcontent for the latest availability
information and additional product content details.
TBD: The Pb-Free/Green conversion plan has not been defined.
Pb-Free (RoHS): TI's terms "Lead-Free" or "Pb-Free" mean semiconductor products that are compatible with the current RoHS requirements for all 6 substances, including the requirement that
lead not exceed 0.1% by weight in homogeneous materials. Where designed to be soldered at high temperatures, TI Pb-Free products are suitable for use in specified lead-free processes.
Pb-Free (RoHS Exempt): This component has a RoHS exemption for either 1) lead-based flip-chip solder bumps used between the die and package, or 2) lead-based die adhesive used between
the die and leadframe. The component is otherwise considered Pb-Free (RoHS compatible) as defined above.
Green (RoHS & no Sb/Br): TI defines "Green" to mean Pb-Free (RoHS compatible), and free of Bromine (Br) and Antimony (Sb) based flame retardants (Br or Sb do not exceed 0.1% by weight
in homogeneous material)
(3) MSL, Peak Temp. - The Moisture Sensitivity Level rating according to the JEDEC industry standard classifications, and peak solder temperature.
(4) There may be additional marking, which relates to the logo, the lot trace code information, or the environmental category on the device.
(5) Multiple Device Markings will be inside parentheses. Only one Device Marking contained in parentheses and separated by a "~" will appear on a device. If a line is indented then it is a continuation
of the previous line and the two combined represent the entire Device Marking for that device.
(6) Lead/Ball Finish - Orderable Devices may have multiple material finish options. Finish options are separated by a vertical ruled line. Lead/Ball Finish values may wrap to two lines if the finish
value exceeds the maximum column width.
PACKAGE OPTION ADDENDUM
www.ti.com 10-Jun-2014
Addendum-Page 3
Important Information and Disclaimer:The information provided on this page represents TI's knowledge and belief as of the date that it is provided. TI bases its knowledge and belief on information
provided by third parties, and makes no representation or warranty as to the accuracy of such information. Efforts are underway to better integrate information from third parties. TI has taken and
continues to take reasonable steps to provide representative and accurate information but may not have conducted destructive testing or chemical analysis on incoming materials and chemicals.
TI and TI suppliers consider certain information to be proprietary, and thus CAS numbers and other limited information may not be available for release.
In no event shall TI's liability arising out of such information exceed the total purchase price of the TI part(s) at issue in this document sold by TI to Customer on an annual basis.
TAPE AND REEL INFORMATION
*All dimensions are nominal
Device Package
Type
Package
Drawing
Pins SPQ Reel
Diameter
(mm)
Reel
Width
W1 (mm)
A0
(mm)
B0
(mm)
K0
(mm)
P1
(mm)
W
(mm)
Pin1
Quadrant
OPA627AU/2K5 SOIC D 8 2500 330.0 12.4 6.4 5.2 2.1 8.0 12.0 Q1
OPA637AU/2K5 SOIC D 8 2500 330.0 12.4 6.4 5.2 2.1 8.0 12.0 Q1
PACKAGE MATERIALS INFORMATION
www.ti.com 26-Jan-2013
Pack Materials-Page 1
*All dimensions are nominal
Device Package Type Package Drawing Pins SPQ Length (mm) Width (mm) Height (mm)
OPA627AU/2K5 SOIC D 8 2500 367.0 367.0 35.0
OPA637AU/2K5 SOIC D 8 2500 367.0 367.0 35.0
PACKAGE MATERIALS INFORMATION
www.ti.com 26-Jan-2013
Pack Materials-Page 2
IMPORTANT NOTICE
Texas Instruments Incorporated and its subsidiaries (TI) reserve the right to make corrections, enhancements, improvements and other
changes to its semiconductor products and services per JESD46, latest issue, and to discontinue any product or service per JESD48, latest
issue. Buyers should obtain the latest relevant information before placing orders and should verify that such information is current and
complete. All semiconductor products (also referred to herein as “components”) are sold subject to TI’s terms and conditions of sale
supplied at the time of order acknowledgment.
TI warrants performance of its components to the specifications applicable at the time of sale, in accordance with the warranty in TI’s terms
and conditions of sale of semiconductor products. Testing and other quality control techniques are used to the extent TI deems necessary
to support this warranty. Except where mandated by applicable law, testing of all parameters of each component is not necessarily
performed.
TI assumes no liability for applications assistance or the design of Buyers’ products. Buyers are responsible for their products and
applications using TI components. To minimize the risks associated with Buyers’ products and applications, Buyers should provide
adequate design and operating safeguards.
TI does not warrant or represent that any license, either express or implied, is granted under any patent right, copyright, mask work right, or
other intellectual property right relating to any combination, machine, or process in which TI components or services are used. Information
published by TI regarding third-party products or services does not constitute a license to use such products or services or a warranty or
endorsement thereof. Use of such information may require a license from a third party under the patents or other intellectual property of the
third party, or a license from TI under the patents or other intellectual property of TI.
Reproduction of significant portions of TI information in TI data books or data sheets is permissible only if reproduction is without alteration
and is accompanied by all associated warranties, conditions, limitations, and notices. TI is not responsible or liable for such altered
documentation. Information of third parties may be subject to additional restrictions.
Resale of TI components or services with statements different from or beyond the parameters stated by TI for that component or service
voids all express and any implied warranties for the associated TI component or service and is an unfair and deceptive business practice.
TI is not responsible or liable for any such statements.
Buyer acknowledges and agrees that it is solely responsible for compliance with all legal, regulatory and safety-related requirements
concerning its products, and any use of TI components in its applications, notwithstanding any applications-related information or support
that may be provided by TI. Buyer represents and agrees that it has all the necessary expertise to create and implement safeguards which
anticipate dangerous consequences of failures, monitor failures and their consequences, lessen the likelihood of failures that might cause
harm and take appropriate remedial actions. Buyer will fully indemnify TI and its representatives against any damages arising out of the use
of any TI components in safety-critical applications.
In some cases, TI components may be promoted specifically to facilitate safety-related applications. With such components, TI’s goal is to
help enable customers to design and create their own end-product solutions that meet applicable functional safety standards and
requirements. Nonetheless, such components are subject to these terms.
No TI components are authorized for use in FDA Class III (or similar life-critical medical equipment) unless authorized officers of the parties
have executed a special agreement specifically governing such use.
Only those TI components which TI has specifically designated as military grade or “enhanced plastic” are designed and intended for use in
military/aerospace applications or environments. Buyer acknowledges and agrees that any military or aerospace use of TI components
which have not been so designated is solely at the Buyer's risk, and that Buyer is solely responsible for compliance with all legal and
regulatory requirements in connection with such use.
TI has specifically designated certain components as meeting ISO/TS16949 requirements, mainly for automotive use. In any case of use of
non-designated products, TI will not be responsible for any failure to meet ISO/TS16949.
Products Applications
Audio www.ti.com/audio Automotive and Transportation www.ti.com/automotive
Amplifiers amplifier.ti.com Communications and Telecom www.ti.com/communications
Data Converters dataconverter.ti.com Computers and Peripherals www.ti.com/computers
DLP® Products www.dlp.com Consumer Electronics www.ti.com/consumer-apps
DSP dsp.ti.com Energy and Lighting www.ti.com/energy
Clocks and Timers www.ti.com/clocks Industrial www.ti.com/industrial
Interface interface.ti.com Medical www.ti.com/medical
Logic logic.ti.com Security www.ti.com/security
Power Mgmt power.ti.com Space, Avionics and Defense www.ti.com/space-avionics-defense
Microcontrollers microcontroller.ti.com Video and Imaging www.ti.com/video
RFID www.ti-rfid.com
OMAP Applications Processors www.ti.com/omap TI E2E Community e2e.ti.com
Wireless Connectivity www.ti.com/wirelessconnectivity
Mailing Address: Texas Instruments, Post Office Box 655303, Dallas, Texas 75265
Copyright © 2014, Texas Instruments Incorporated
For free samples & the latest literature: http://www.maxim-ic.com, or phone 1-800-998-8800.
For small orders, phone 1-800-835-8769.
General Description
The MAX4661/MAX4662/MAX4663 quad analog switches
feature low on-resistance of 2.5½ max. On-resistance is
matched between switches to 0.5W max and is flat
(0.5W max) over the specified signal range. Each
switch can handle Rail-to-Rail® analog signals. Offleakage
current is only 5nA max at TA = +85°C. These
analog switches are ideal in low-distortion applications
and are the preferred solution over mechanical relays in
automatic test equipment or applications where current
switching is required. They have lower power requirements,
use less board space, and are more reliable
than mechanical relays.
The MAX4661 has four normally closed (NC) switches,
and the MAX4662 has four normally open (NO) switches.
The MAX4663 has two NC and two NO switches, and features
guaranteed break-before-make switching.
These devices operate from a single +4.5V to +36V supply
or from dual ±4.5V to ±20V supplies. A separate logic
supply pin guarantees TTL/CMOS-logic compatibility
when operating across the entire supply voltage range.
Applications
Reed Relay Replacement Avionics
Test Equipment ADC Systems
Communication Systems Sample-and-Hold Circuits
PBX, PABX Systems Data Acquisition Systems
Audio-Signal Routing
Features
© Low On-Resistance (2.5W max)
© Guaranteed RON Match Between Channels
(0.5W max)
© Guaranteed RON Flatness over Specified Signal
Range (0.5W max)
© Rail-to-Rail Signal Handling
© Guaranteed Break-Before-Make (MAX4663)
© > 2kV ESD Protection per Method 3015.7
© +4.5V to +36V Single-Supply Operation
±4.5V to ±20V Dual-Supply Operation
© TTL/CMOS-Compatible Control Inputs
MAX4661/MAX4662/MAX4663
2.5W, Quad, SPST,
CMOS Analog Switches
________________________________________________________________ Maxim Integrated Products 1
19-1516; Rev 0; 7/99
PART
MAX4661CAE
MAX4661CWE
MAX4661CPE 0°C to +70°C
0°C to +70°C
0°C to +70°C
TEMP. RANGE PIN-PACKAGE
16 SSOP
16 Wide SO
16 Plastic DIP
Ordering Information continued at end of data sheet.
Ordering Information
MAX4661EAE -40°C to +85°C 16 SSOP
MAX4661EWE -40°C to +85°C 16 Wide SO
MAX4661EPE -40°C to +85°C 16 Plastic DIP
SWITCHES SHOWN FOR LOGIC “0” INPUT
SSOP/SO/DIP
MAX4662
LOGIC SWITCH
0
1
OFF
ON
TOP VIEW
SSOP/SO/DIP
MAX4661
LOGIC SWITCH
0
1
ON
OFF
SSOP/SO/DIP
MAX4663
LOGIC SWITCHES
1, 4
0
1
OFF
ON
SWITCHES
2, 3
ON
OFF
16
15
14
13
12
11
10
9
1
2
3
4
5
6
7
8
IN2
COM2
NC2
V- V+
NO1
COM1
IN1
MAX4663 VL
NC3
COM3
IN4 IN3
COM4
NO4
GND
16
15
14
13
12
11
10
9
1
2
3
4
5
6
7
8
IN2
COM2
NC2
V- V+
NC1
COM1
IN1
MAX4661 VL
NC3
COM3
IN4 IN3
COM4
NC4
GND
16
15
14
13
12
11
10
9
1
2
3
4
5
6
7
8
IN2
COM2
NO2
V- V+
NO1
COM1
IN1
MAX4662 VL
NO3
COM3
IN4 IN3
COM4
NO4
GND
Pin Configurations/Functional Diagrams/Truth Tables
Rail-to-Rail is a registered trademark of Nippon Motorola, Ltd.
MAX4661/MAX4662/MAX4663
2.5W, Quad, SPST,
CMOS Analog Switches
2 _______________________________________________________________________________________
ABSOLUTE MAXIMUM RATINGS
Stresses beyond those listed under “Absolute Maximum Ratings” may cause permanent damage to the device. These are stress ratings only, and functional
operation of the device at these or any other conditions beyond those indicated in the operational sections of the specifications is not implied. Exposure to
absolute maximum rating conditions for extended periods may affect device reliability.
V+ to GND ..............................................................-0.3V to +44V
V- to GND ..............................................................+0.3V to -44V
V+ to V-...................................................................-0.3V to +44V
VL to GND .......................................(GND - 0.3V) to (V+ + 0.3V)
All Other Pins to GND (Note 1) .............(V- - 0.3V) to (V+ + 0.3V)
Continuous Current (COM_, NO_, NC_) ........................±200mA
Peak Current (COM_, NO_, NC_)
(pulsed at 1ms, 10% duty cycle)................................ ±300mA
Continuous Power Dissipation (TA = +70°C)
SSOP (derate 7.1mW/°C above +70°C) .......................571mW
Wide SO (derate 9.52mW/°C above +70°C).................762mW
Plastic DIP (derate 10.53mW/°C above +70°C) ...........842mW
Operating Temperature Ranges
MAX466_C_E ......................................................0°C to +70°C
MAX466_E_E ....................................................-40°C to +85°C
Storage Temperature Range .............................-65°C to +150°C
Lead Temperature (soldering, 10sec) .............................+300°C
-5 5
-5 5
-20 20
TA = TMIN to TMAX
V
TA = +25°C
V- V+
VCOM_,
VNO_, VNC_
Input Voltage Range (Note 3)
IN_ = 0.8V, all others = 2.4V
IN_ = 2.4V, all others = 0.8V
ICOM_ = 10mA,
VNO_ or VNC_ = ±10V
TA = +25°C
TA = +25°C
TA = +25°C
CONDITIONS
Logic Input Voltage Low VIN_L 0.8
½
0.1 0.5
ÆRON
COM_ to NO_ or NC_
On-Resistance Match Between
Channels (Notes 3, 4)
½
1.7 2.5
RON
COM_ to NO or NC_
On-Resistance
Logic Input Voltage High VIN_H 2.4 V
IIN_L -0.5 0.001 0.5
Input Current with Input Voltage
Low
IIN_H -0.5 0.001 0.5 μA
Input Current with Input Voltage
High
½
0.1 0.5
RFLAT(ON)
COM_ to NO_ or NC_
On-Resistance Flatness
(Notes 3, 5)
nA
-0.5 0.01 0.5
INO_, INC_
Off-Leakage Current
(NO_ or NC_) (Note 6)
nA
-0.5 0.01 0.5
ICOM_(OFF)
COM Off-Leakage Current
(Note 6)
nA
-1 0.01 1
ICOM_(ON)
COM On-Leakage Current
(Note 6)
PARAMETER SYMBOL MIN TYP MAX UNITS
ICOM_ = 10mA,
VNO_ or VNC_= ±10V
ICOM_ = 10mA; VNO_
or VNC_ = -5V, 0, 5V
TA = +25°C
VCOM_ = ±10V,
VNO_ or VNC_= –+
10V
VCOM_ = ±10V,
VNO_ or VNC_ = –+
10V
VCOM_ = ±10V,
VNO_ or VNC_= ±10V
or floating
TA = +25°C
TA = TMIN to TMAX
2.7
0.6
TA = TMIN to TMAX 0.6
TA = TMIN to TMAX
TA = TMIN to TMAX
TA = TMIN to TMAX
ELECTRICAL CHARACTERISTICS—Dual Supplies
(V+ = +15V, V- = -15V, VL = +5V, VIN_H = +2.4V, VIN_L = +0.8V, TA = TMIN to TMAX, unless otherwise noted. Typical values are at
TA = +25°C.) (Note 2)
μA
V
ANALOG SWITCH
LOGIC INPUT
Note 1: Signals on NC_, NO_, COM_, or IN_ exceeding V+ or V- will be clamped by internal diodes. Limit forward diode current to maximum
current rating.
MAX4661/MAX4662/MAX4663
2.5W, Quad, SPST,
CMOS Analog Switches
_______________________________________________________________________________________ 3
VIN = 0 or 5V μA
TA = +25°C
-0.5 0.001 0.5
Positive Supply Current I+
TA = +25°C
f = 1MHz, Figure 7
RL = 50½, CL = 5pF, f = 1MHz,
Figure 6
TA = TMIN to TMAX -5 5
RL = 50½, CL = 5pF, f = 1MHz,
Figure 5
VCOM_ = ±10V, Figure 3, TA = +25°C
VIN = 0 or 5V
TA = +25°C
f = 1MHz, Figure 7
CONDITIONS
Power-Supply Range ±4.5 ±20.0 V
pF
dB
On-Capacitance CCOM f = 1MHz, Figure 8 250 pF
COM_ Off-Capacitance CCOM 55
-0.5 0.001 0.5
Logic Supply Current IL
-0.5 0.001 0.5
Negative Supply Current INC_
or NO_ Capacitance COFF pF
Crosstalk (Note 8) VCT -59
Off-Isolation (Note 7) VISO -56 dB
tOPEN 5 30 ns
Break-Before-Make Time
(MAX4663 only)
-0.5 0.001 0.5
Ground Current IGND
130 275
100 175
PARAMETER SYMBOL MIN TYP MAX UNITS
VIN = 0 or 5V
VIN = 0 or 5V
TA = +25°C
TA = TMIN to TMAX
-5 5
-5 5
TA = TMIN to TMAX -5 5
ELECTRICAL CHARACTERISTICS—Dual Supplies (continued)
(V+ = +15V, V- = -15V, VL = +5V, VIN_H = +2.4V, VIN_L = +0.8V, TA = TMIN to TMAX, unless otherwise noted. Typical values are at
TA = +25°C.) (Note 2)
μA
μA
μA
TA = TMIN to TMAX
55
ns
400
Turn-On Time tON
VCOM_ = ±10V,
Figure 2
ns
300
Turn-Off Time tOFF
VCOM_ = ±10V,
Figure 2
CL = 1.0nF, VGEN = 0, RGEN = 0,
Figure 4
Charge Injection Q 300 pC
POWER SUPPLY
SWITCH DYNAMIC CHARACTERISTICS
TA = +25°C
TA = TMIN to TMAX
TA = +25°C
TA = TMIN to TMAX
MAX4661/MAX4662/MAX4663
2.5W, Quad, SPST,
CMOS Analog Switches
4 _______________________________________________________________________________________
-5 5
-5 5
-20 20
TA = TMIN to TMAX
V
TA = +25°C
GND V+
VCOM_, VNO_,
VNC_
Input Voltage Range (Note 3)
IN_ = 0.8V, all others = 2.4V
+4.5 +36.0
IN_ = 2.4V, all others = 0.8V
ICOM_ = 10mA,
VNO_ or VNC_ = 10V
TA = +25°C
TA = +25°C
TA = +25°C
CONDITIONS
Power-Supply Range
VIN = 0 or 5V
VIN = 0 or 5V
-0.5 0.001 0.5
Logic Supply Current IL
μA
-0.5 0.001 0.5
Positive Supply Current I+
V
μA
VIN = 0 or 5V
-0.5 0.001 0.5
Ground Current IGND μA
TA = +25°C
TA = +25°C
TA = TMIN to TMAX
TA = +25°C
TA = TMIN to TMAX
TA = TMIN to TMAX
-5 5
-5 5
-5 5
Logic Input Voltage Low VIN_L 0.8
½
0.03 0.4
ÆRON
COM_ to NO_ or NC_
On-Resistance Match Between
Channels (Notes 3, 4)
½
3 4
RON
COM_ to NO or NC_
On-Resistance
Logic Input Voltage High VIN_H 2.4 V
IIN_L -0.5 0.001 0.5
Input Current with Input Voltage
Low
IIN_H -0.5 0.001 0.5 μA
Input Current with Input Voltage
High
½
0.1 0.7
RFLAT(ON)
COM_ to NO_ or NC_
On-Resistance Flatness
(Notes 3, 5)
nA
I -0.5 0.01 0.5 NO_
INC_
Off-Leakage Current
(NO_ or NC_) (Notes 6, 9)
nA
-0.5 0.01 0.5
ICOM_(OFF)
COM Off-Leakage Current
(Notes 6, 9)
nA
-1 0.01 1
ICOM_(ON)
COM On-Leakage Current
(Notes 6, 9)
PARAMETER SYMBOL MIN TYP MAX UNITS
ICOM_ = 10mA,
VNO_ or = VNC_= 10V
ICOM_ = 10mA; VNO_
or VNC_ = 3V, 6V, 9V
TA = +25°C
VCOM_ = 1V, 10V;
VNO_ or VNC_ = 10V,
1V
VNO_ or VNC_ = 10V,
1V; VCOM_ = 1V, 10V
VCOM_ = 1V ,10V;
VNO_ or VNC_ = 1V,
10V, or floating
TA = +25°C
TA = TMIN to TMAX
5
0.5
TA = TMIN to TMAX 0.8
TA = TMIN to TMAX
TA = TMIN to TMAX
TA = TMIN to TMAX
ELECTRICAL CHARACTERISTICS—Single Supply
(V+ = +12V, V- = 0, VL = +5V, VIN_H = +2.4V, VIN_L = +0.8V, TA = TMIN to TMAX, unless otherwise noted. Typical values are at
TA = +25°C.) (Note 2)
μA
V
ANALOG SWITCH
LOGIC INPUT
POWER SUPPLY
MAX4661/MAX4662/MAX4663
2.5W, Quad, SPST,
CMOS Analog Switches
_______________________________________________________________________________________ 5
ELECTRICAL CHARACTERISTICS—Single Supply (continued)
(V+ = +12V, V- = 0, VL = +5V, VIN_H = +2.4V, VIN_L = +0.8V, TA = TMIN to TMAX, unless otherwise noted. Typical values are at
TA = +25°C.) (Note 2)
Note 2: The algebraic convention, where the most negative value is a minimum and the most positive value a maximum, is used in
this data sheet.
Note 3: Guaranteed by design.
Note 4: DRON = RON(MAX) - RON(MIN).
Note 5: Flatness is defined as the difference between the maximum and minimum value of on-resistance as measured over the
specified analog signal range.
Note 6: Leakage parameters are 100% tested at maximum-rated hot temperature and guaranteed by correlation at +25°C.
Note 7: Off-isolation = 20log10 [VCOM / (VNC or VNO)], VCOM = output, VNC or VNO = input to off switch.
Note 8: Between any two switches.
Note 9: Leakage testing at single supply is guaranteed by testing with dual supplies.
200 400
On-Capacitance CCOM f = 1MHz, Figure 8 140 pF
COM Off-Capacitance CCOM f = 1MHz, Figure 7 85 pF
NC_ or NO_ Capacitance COFF f = 1MHz, Figure 7 85 pF
RL = 50½, CL = 5pF, f = 1MHz,
Figure 6
VCOM_ = 10V, Figure 3, TA = +25°C
PARAMETER SYMBOL MIN TYP MAX UNITS
Crosstalk (Note 8) VCT -60 dB
Break-Before-Make Time
(MAX4663 only) (Note 3)
tOPEN 5 125 ns
100 250
CONDITIONS
Turn-On Time (Note 3) tON 500
ns
VCOM_ = 10V,
Figure 2
VCOM_ = 10V,
Figure 2
Turn-Off Time (Note 3) tOFF 350
ns
TA = +25°C
TA = TMIN to TMAX
TA = +25°C
TA = TMIN to TMAX
CL = 1.0nF, VGEN = 0, RGEN = 0,
Figure 4
Charge Injection Q 20 pC
SWITCH DYNAMIC CHARACTERISTICS
MAX4661/MAX4662/MAX4663
2.5W, Quad, SPST,
CMOS Analog Switches
6 _______________________________________________________________________________________
Typical Operating Characteristics
(TA = +25°C, unless otherwise noted.)
0
1.0
0.5
2.0
1.5
3.0
2.5
3.5
4.5
4.0
5.0
-20 -15 -10 -5 0 5 10 15 20
ON-RESISTANCE vs. VCOM
(DUAL SUPPLIES)
MAX4661/2/3-01
VCOM (V)
RON (W)
V+, V- = ±5V
V+, V- = ±15V
V+, V- = ±20V
0
0.50
0.25
1.00
0.75
1.50
1.25
1.75
2.25
2.00
2.50
-15 -10 -5 0 5 10 15
ON-RESISTANCE vs. VCOM AND
TEMPERATURE (DUAL SUPPLIES)
MAX4661/2/3-02
VCOM (V)
RON (W)
TA = +85°C
TA = +25°C
V+, V- = ±15V
TA = -40°C
0
3
2
1
4
5
6
7
8
9
10
0 2 4 6 8 10 12 14 16 18 20 22 24
ON-RESISTANCE vs. VCOM
(SINGLE SUPPLY)
MAX4661/2/3-03
VCOM (V)
RON (W)
V+ = 5V
V+ = 12V
V+ = 24V
0
1.50
1.00
0.50
2.00
2.50
3.50
3.00
4.00
0 1 2 3 4 5 6 7 8 9 10 11 12
ON-RESISTANCE vs. VCOM AND
TEMPERATURE (SINGLE SUPPLY)
MAX4661/2/3-04
VCOM (V)
RON (W)
TA = +85°C
TA = +25°C
TA = -40°C
V+ = +12V
V- = GND
0.1m
0.01
1m
10
1
0.1
1k
10k
100
100k
-40 -20 0 20 40 60 80 100
ON/OFF-LEAKAGE CURRENT
vs. TEMPERATURE
MAX4661/2/3-05
TEMPERATURE (°C)
LEAKAGE (pA)
ON-LEAKAGE
OFF-LEAKAGE
V+ = +15V
V- = -15V
-200
0
-100
200
100
300
400
-20 -15 -10 -5 0 5 10 15 20
CHARGE INJECTION
vs. VCOM
MAX4661/2/3-06
VCOM (V)
Q (pC)
V- = -15V
V+ = +15V
V- = GND
V+ = 12V
0.1
I+
I-
0.01
0.001
100
10
1
10k
100k
1k
-40 -20 0 20 40 60 80 100
POWER-SUPPLY CURRENT
vs. TEMPERATURE
MAX4661/2/3-07
TEMPERATURE (°C)
I+, I- (nA)
V+ = +15V
V- = -15V -10
-100
0.1 1 10 100
FREQUENCY RESPONSE
-70
-90
-30
-50
0
-60
-80
-20
-40
MAX4661/2/3-08
FREQUENCY (MHz)
LOSS (dB)
90
180
-720
-450
-630
-90
-270
-360
-540
-0
-180
PHASE (degrees)
OFF-ISOLATION
ON-PHASE
ON-RESPONSE
V+ = +15V
V- = -15V
INPUT = OdBm
50W IN AND OUT
MAX4661/MAX4662/MAX4663
2.5W, Quad, SPST,
CMOS Analog Switches
_______________________________________________________________________________________ 7
NAME FUNCTION
MAX4661
1, 16, 9, 8
IN1, IN2,
IN3, IN4
Logic-Control Digital Inputs
2, 15,
10, 7
COM1, COM2,
COM3, COM4
Analog Switch Common Terminals
3, 14, 11, 6
NC1, NC2,
NC3, NC4
Analog Switch Normally Closed Terminals
4 VNegative
Analog Supply-Voltage Input. Connect to GND for singlesupply
operation.
— NC2, NC3 Analog Switch Normally Closed Terminals
— NO1, NO4 Analog Switch Normally Open Terminals
—
NO1, NO2,
NO3, NO4
Analog Switch Normally Open Terminals
13 V+ Positive Analog Supply Input
12 VL Logic-Supply Input
5 GND Ground
Pin Description
MAX4662
1, 16, 9, 8
2, 15,
10, 7
—
4
—
—
3, 14, 11, 6
13
12
5
MAX4663
1, 16, 9, 8
PIN
2, 15,
10, 7
—
4
14, 11
3, 6
—
13
12
5
Applications Information
Overvoltage Protection
Proper power-supply sequencing is recommended for
all CMOS devices. Do not exceed the absolute maximum
ratings because stresses beyond the listed ratings
can cause permanent damage to the devices.
Always sequence V+ on first, then V-, followed by the
logic inputs, NO, or COM. If power-supply sequencing
is not possible, add two small-signal diodes (D1, D2)
in series with the supply pins and a Schottky diode
between V+ and VL for overvoltage protection (Figure
1). Adding diodes reduces the analog signal range to
one diode drop below V+ and one diode drop above V-,
but does not affect the devices’ low switch resistance
and low leakage characteristics. Device operation is
unchanged, and the difference between V+ and Vshould
not exceed 44V.
Off-Isolation at High Frequencies
In 50½ systems, the high-frequency on-response of
these parts extends from DC to above 100MHz with a
typical loss of -2dB. When the switch is turned off, however,
it behaves like a capacitor and off-isolation
decreases with increasing frequency. (Above 300MHz,
the switch actually passes more signal turned off than
turned on.) This effect is more pronounced with higher
source and load impedances.
Above 5MHz, circuit board layout becomes critical and
it becomes difficult to characterize the response of the
switch independent of the circuit. The graphs shown in
the Typical Operating Characteristics were taken using
a 50½ source and load connected with BNC connectors
to a circuit board deemed “average”; that is,
designed with isolation in mind, but not using stripline
or other special RF circuit techniques. For critical applications
above 5MHz, use the MAX440, MAX441, and
MAX442, which are fully characterized up to 160MHz.
COM_
VV+
VL
NO_
* INTERNAL PROTECTION DIODES
D2
D1
-15V
+15V
MAX4661
MAX4662
MAX4663
*
*
*
*
Figure 1. Overvoltage Protection Using External Blocking
Diodes
MAX4661/MAX4662/MAX4663
2.5W, Quad, SPST,
CMOS Analog Switches
8 _______________________________________________________________________________________
50%
0.9 · V0UT1
+3V
0V
0V
LOGIC
INPUT
SWITCH
OUTPUT 2
(VOUT2)
0V
0.9 · VOUT2
tD tD
LOGIC
INPUT
V-
-15V
RL2
GND
CL INCLUDES FIXTURE AND STRAY CAPACITANCE.
COM2
IN1, 2
COM1
VOUT2
V+
+15V
CL2
VCOM1
RL1
VOUT1
CL1
RL = 100W
CL = 35pF
NO
NC SWITCH
OUTPUT 1
(VOUT1)
MAX4663
VCOM2
Figure 3. Break-Before-Make Interval (MAX4663 only)
tr < 20ns
tf < 20ns
50%
0
LOGIC
INPUT
V-
-15V
RL
100W
NO_
OR NC_
GND
CL INCLUDES FIXTURE AND STRAY CAPACITANCE.
VO = VCOM ( RL RL + RON)
SWITCH
INPUT
IN_
+3V
tOFF
0
COM_
SWITCH
OUTPUT
0.9V0 0.9V0
tON
VO
SWITCH
OUTPUT
LOGIC
INPUT
LOGIC INPUT WAVEFORMS INVERTED FOR SWITCHES
THAT HAVE THE OPPOSITE LOGIC SENSE.
VL V+
CL
35pF
+5V +15V
VCOM_ VO
0
REPEAT TEST FOR EACH SWITCH. FOR LOAD
CONDITIONS, SEE Electrical Characteristics.
MAX4661
MAX4662
MAX4663
Figure 2. Switching-Time Test Circuit
MAX4661/MAX4662/MAX4663
2.5W, Quad, SPST,
CMOS Analog Switches
_______________________________________________________________________________________ 9
VGEN
GND
NC OR
NO
CL
VO
-15V
VV+
VO
VIN
OFF
ON
OFF
DVO
Q = (DVO)(CL)
COM
+5V
VIN DEPENDS ON SWITCH CONFIGURATION;
INPUT POLARITY DETERMINED BY SENSE OF SWITCH.
OFF
ON
OFF
VIN
VIN = +3V
+15V
RGEN
IN
VL
MAX4661
MAX4662
MAX4663
Figure 4. Charge-Injection Test Circuit
IN
0 OR 3.0V
SIGNAL
GENERATOR 0dBm
+15V
VL
ANALYZER NC OR NO
RL
GND
COM
-15V
V-
+5V
COM
V+
MAX4661
MAX4662
MAX4663
Figure 5. Off-Isolation Test Circuit
SIGNAL
GENERATOR 0dBm
+15V
ANALYZER N_2
RL
GND
COM1
V-
-15V
3.0V
IN1
N_1 VL 50W
COM2
+5V
IN2
0 OR 3.0V
N.C.
V+
MAX4661
MAX4662
MAX4663
Figure 6. Crosstalk Test Circuit
MAX4661/MAX4662/MAX4663
2.5W, Quad, SPST,
CMOS Analog Switches
10 ______________________________________________________________________________________
CAPACITANCE
METER
NC OR NO
COM
GND V-
-15V
IN 0 OR
3.0V
+15V
VL
+5V
f = 1MHz
V+
MAX4661
MAX4662
MAX4663
Figure 7. Switch Off-Capacitance Test Circuit
CAPACITANCE
METER
NC OR NO
COM
GND V-
-15V
IN 0 OR
3.0V
+15V
VL
+5V
f = 1MHz
V+
MAX4661
MAX4662
MAX4663
Figure 8. Switch On-Capacitance Test Circuit
Chip Information
TRANSISTOR COUNT: 108
Ordering Information (continued)
PART TEMP. RANGE PIN-PACKAGE
MAX4662CAE 0°C to +70°C 16 SSOP
MAX4662CWE 0°C to +70°C 16 Wide SO
MAX4662CPE 0°C to +70°C 16 Plastic DIP
MAX4662EAE -40°C to +85°C 16 SSOP
MAX4662EWE -40°C to +85°C 16 Wide SO
MAX4662EPE -40°C to +85°C 16 Plastic DIP
MAX4663CAE 0°C to +70°C 16 SSOP
MAX4663CWE 0°C to +70°C 16 Wide SO
MAX4663CPE 0°C to +70°C 16 Plastic DIP
MAX4663EAE -40°C to +85°C 16 SSOP
MAX4663EWE -40°C to +85°C 16 Wide SO
MAX4663EPE -40°C to +85°C 16 Plastic DIP
MAX4661/MAX4662/MAX4663
2.5W, Quad, SPST,
CMOS Analog Switches
______________________________________________________________________________________ 11
Package Information
SSOP.EPS
MAX4661/MAX4662/MAX46663
2.5W, Quad, SPST,
CMOS Analog Switches
Maxim cannot assume responsibility for use of any circuitry other than circuitry entirely embodied in a Maxim product. No circuit patent licenses are
implied. Maxim reserves the right to change the circuitry and specifications without notice at any time.
12 ____________________Maxim Integrated Products, 120 San Gabriel Drive, Sunnyvale, CA 94086 408-737-7600
© 1999 Maxim Integrated Products Printed USA is a registered trademark of Maxim Integrated Products.
Package Information (continued)
SOICW.EPS
Copyright © 2010 Future Technology Devices International Limited 1
Document No.: FT_000053
FT232R USB UART IC Datasheet Version 2.10
Clearance No.: FTDI# 38
Future Technology Devices International Ltd.
FT232R USB UART IC
The FT232R is a USB to serial UART interface with the following advanced features: Single chip USB to asynchronous serial data transfer interface. Entire USB protocol handled on the chip. No USB specific firmware programming required. Fully integrated 1024 bit EEPROM storing device descriptors and CBUS I/O configuration. Fully integrated USB termination resistors. Fully integrated clock generation with no external crystal required plus optional clock output selection enabling a glue-less interface to external MCU or FPGA. Data transfer rates from 300 baud to 3 Mbaud (RS422, RS485, RS232 ) at TTL levels. 128 byte receive buffer and 256 byte transmit buffer utilising buffer smoothing technology to allow for high data throughput. FTDI‟s royalty-free Virtual Com Port (VCP) and Direct (D2XX) drivers eliminate the requirement for USB driver development in most cases. Unique USB FTDIChip-ID™ feature. Configurable CBUS I/O pins. Transmit and receive LED drive signals. UART interface support for 7 or 8 data bits, 1 or 2 stop bits and odd / even / mark / space / no parity FIFO receive and transmit buffers for high data throughput. Synchronous and asynchronous bit bang interface options with RD# and WR# strobes. Device supplied pre-programmed with unique USB serial number. Supports bus powered, self powered and high-power bus powered USB configurations. Integrated +3.3V level converter for USB I/O. Integrated level converter on UART and CBUS for interfacing to between +1.8V and +5V logic. True 5V/3.3V/2.8V/1.8V CMOS drive output and TTL input. Configurable I/O pin output drive strength. Integrated power-on-reset circuit. Fully integrated AVCC supply filtering - no external filtering required. UART signal inversion option. +3.3V (using external oscillator) to +5.25V (internal oscillator) Single Supply Operation. Low operating and USB suspend current. Low USB bandwidth consumption. UHCI/OHCI/EHCI host controller compatible. USB 2.0 Full Speed compatible. -40°C to 85°C extended operating temperature range. Available in compact Pb-free 28 Pin SSOP and QFN-32 packages (both RoHS compliant).
Neither the whole nor any part of the information contained in, or the product described in this manual, may be adapted or reproduced in any material or electronic form without the prior written consent of the copyright holder. This product and its documentation are supplied on an as-is basis and no warranty as to their suitability for any particular purpose is either made or implied. Future Technology Devices International Ltd will not accept any claim for damages howsoever arising as a result of use or failure of this product. Your statutory rights are not affected. This product or any variant of it is not intended for use in any medical appliance, device or system in which the failure of the product might reasonably be expected to result in personal injury. This document provides preliminary information that may be subject to change without notice. No freedom to use patents or other intellectual property rights is implied by the publication of this document. Future Technology Devices International Ltd, Unit 1, 2 Seaward Place, Centurion Business Park, Glasgow G41 1HH United Kingdom. Scotland Registered Company Number: SC136640
Copyright © 2010 Future Technology Devices International Limited 2
Document No.: FT_000053
FT232R USB UART IC Datasheet Version 2.10
Clearance No.: FTDI# 38
1 Typical Applications USB to RS232/RS422/RS485 Converters Upgrading Legacy Peripherals to USB Cellular and Cordless Phone USB data transfer cables and interfaces Interfacing MCU/PLD/FPGA based designs to USB USB Audio and Low Bandwidth Video data transfer PDA to USB data transfer USB Smart Card Readers USB Instrumentation USB Industrial Control USB MP3 Player Interface USB FLASH Card Reader and Writers Set Top Box PC - USB interface USB Digital Camera Interface USB Hardware Modems USB Wireless Modems USB Bar Code Readers USB Software and Hardware Encryption Dongles
1.1 Driver Support
Royalty free VIRTUAL COM PORT
(VCP) DRIVERS for... Windows 98, 98SE, ME, 2000, Server 2003, XP and Server 2008 Windows 7 32,64-bit Windows XP and XP 64-bit Windows Vista and Vista 64-bit Windows XP Embedded Windows CE 4.2, 5.0 and 6.0 Mac OS 8/9, OS-X Linux 2.4 and greater
Royalty free D2XX Direct Drivers
(USB Drivers + DLL S/W Interface) Windows 98, 98SE, ME, 2000, Server 2003, XP and Server 2008 Windows 7 32,64-bit Windows XP and XP 64-bit Windows Vista and Vista 64-bit Windows XP Embedded Windows CE 4.2, 5.0 and 6.0 Linux 2.4 and greater
The drivers listed above are all available to download for free from FTDI website (www.ftdichip.com). Various 3rd party drivers are also available for other operating systems - see FTDI website (www.ftdichip.com) for details.
For driver installation, please refer to http://www.ftdichip.com/Documents/InstallGuides.htm
1.2 Part Numbers Part Number Package
FT232RQ-xxxx
32 Pin QFN
FT232RL-xxxx
28 Pin SSOP
Note: Packing codes for xxxx is:
- Reel: Taped and Reel, (SSOP is 2,000pcs per reel, QFN is 6,000pcs per reel).
- Tube: Tube packing, 47pcs per tube (SSOP only)
- Tray: Tray packing, 490pcs per tray (QFN only)
For example: FT232RQ-Reel is 6,000pcs taped and reel packing
Copyright © 2010 Future Technology Devices International Limited 3
Document No.: FT_000053
FT232R USB UART IC Datasheet Version 2.10
Clearance No.: FTDI# 38
1.3 USB Compliant
The FT232R is fully compliant with the USB 2.0 specification and has been given the USB-IF Test-ID (TID) 40680004 (Rev B) and 40770018 (Rev C).
Copyright © 2010 Future Technology Devices International Limited 4
Document No.: FT_000053
FT232R USB UART IC Datasheet Version 2.10
Clearance No.: FTDI# 38
2 FT232R Block Diagram
Figure 2.1 FT232R Block Diagram
For a description of each function please refer to Section 4.
x4 ClockMultiplierUARTFIFO ControllerSerial InterfaceEngine( SIE )USBProtocol EngineBaud RateGeneratorUART ControllerwithProgrammableSignal Inversion3.3 VoltLDORegulatorUSBTransceiverwithIntegratedSeriesResistorsand 1.5KPull-upUSB DPLLInternal12MHzOscillator48MHz48MHzOCSI(optional)OSCO(optional)USBDPUSBDM3V3OUTVCCDBUS0DBUS1DBUS2DBUS3DBUS4DBUS5DBUS6DBUS7CBUS0CBUS2CBUS3SLEEP#RESET#TESTGNDResetGenerator3V3OUTCBUS1FIFO RX BufferFIFO TX BufferInternalEEPROMTo USB Transeiver CellCBUS4
Copyright © 2010 Future Technology Devices International Limited 5
Document No.: FT_000053
FT232R USB UART IC Datasheet Version 2.10
Clearance No.: FTDI# 38
Table of Contents
1 Typical Applications ........................................................................ 2
1.1 Driver Support .................................................................................... 2
1.2 Part Numbers...................................................................................... 2
Note: Packing codes for xxxx is: .................................................................. 2
1.3 USB Compliant .................................................................................... 3
2 FT232R Block Diagram .................................................................... 4
3 Device Pin Out and Signal Description ............................................ 7
3.1 28-LD SSOP Package .......................................................................... 7
3.2 SSOP Package Pin Out Description ...................................................... 7
3.3 QFN-32 Package ............................................................................... 10
3.4 QFN-32 Package Signal Description .................................................. 10
3.5 CBUS Signal Options ......................................................................... 13
4 Function Description ..................................................................... 14
4.1 Key Features ..................................................................................... 14
4.2 Functional Block Descriptions ........................................................... 15
5 Devices Characteristics and Ratings .............................................. 17
5.1 Absolute Maximum Ratings............................................................... 17
5.2 DC Characteristics............................................................................. 18
5.3 EEPROM Reliability Characteristics ................................................... 21
5.4 Internal Clock Characteristics ........................................................... 21
6 USB Power Configurations ............................................................ 23
6.1 USB Bus Powered Configuration ...................................................... 23
6.2 Self Powered Configuration .............................................................. 24
6.3 USB Bus Powered with Power Switching Configuration .................... 25
6.4 USB Bus Powered with Selectable External Logic Supply .................. 26
7 Application Examples .................................................................... 27
7.1 USB to RS232 Converter ................................................................... 27
7.2 USB to RS485 Coverter ..................................................................... 28
7.3 USB to RS422 Converter ................................................................... 29
7.4 USB to MCU UART Interface .............................................................. 30
7.5 LED Interface .................................................................................... 31
7.6 Using the External Oscillator ............................................................ 32
8 Internal EEPROM Configuration .................................................... 33
9 Package Parameters ..................................................................... 35
9.1 SSOP-28 Package Dimensions .......................................................... 35
Copyright © 2010 Future Technology Devices International Limited 6
Document No.: FT_000053
FT232R USB UART IC Datasheet Version 2.10
Clearance No.: FTDI# 38
9.2 QFN-32 Package Dimensions ............................................................ 36
9.3 QFN-32 Package Typical Pad Layout ................................................. 37
9.4 QFN-32 Package Typical Solder Paste Diagram ................................. 37
9.5 Solder Reflow Profile ........................................................................ 38
10 Contact Information ................................................................... 39
Appendix A – References ........................................................................... 40
Appendix B - List of Figures and Tables ..................................................... 41
Appendix C - Revision History .................................................................... 43
Copyright © 2010 Future Technology Devices International Limited 7
Document No.: FT_000053
FT232R USB UART IC Datasheet Version 2.10
Clearance No.: FTDI# 38
3 Device Pin Out and Signal Description
3.1 28-LD SSOP Package
Figure 3.1 SSOP Package Pin Out and Schematic Symbol
3.2 SSOP Package Pin Out Description
Note: The convention used throughout this document for active low signals is the signal name followed by a # Pin No. Name Type Description
15
USBDP
I/O
USB Data Signal Plus, incorporating internal series resistor and 1.5kΩ pull up resistor to 3.3V.
16
USBDM
I/O
USB Data Signal Minus, incorporating internal series resistor.
Table 3.1 USB Interface Group
Pin No. Name Type Description
4
VCCIO
PWR
+1.8V to +5.25V supply to the UART Interface and CBUS group pins (1...3, 5, 6, 9...14, 22, 23). In USB bus powered designs connect this pin to 3V3OUT pin to drive out at +3.3V levels, or connect to VCC to drive out at 5V CMOS level. This pin can also be supplied with an external +1.8V to +2.8V supply in order to drive outputs at lower levels. It should be noted that in this case this supply should originate from the same source as the supply to VCC. This means that in bus powered designs a regulator which is supplied by the +5V on the USB bus should be used.
7, 18, 21
GND
PWR
Device ground supply pins
USBDPUSBDM3V3OUTGNDRESET#VCCGNDNCAGNDTESTOSCIOSCOCBUS1CBUS0TXDRTS#RXDDTR#VCCIORI#GNDNCDSR#DCD#CTS#CBUS4CBUS2CBUS31141528FT232RLAGNDGNDGNDGNDTEST2571821263V3OUTVCCIO417NCRESET#NC24198TXDRXDRTS#CTS#DTR#DSR#DCD#RI#1531129106CBUS0CBUS3CBUS2CBUS123221314201615USBDPUSBDMVCCOSCI27OSCO28CBUS412FTDIFT232RLYYXX-AXXXXXXXXXXXX
Copyright © 2010 Future Technology Devices International Limited 8
Document No.: FT_000053
FT232R USB UART IC Datasheet Version 2.10
Clearance No.: FTDI# 38
Pin No. Name Type Description
17
3V3OUT
Output
+3.3V output from integrated LDO regulator. This pin should be decoupled to ground using a 100nF capacitor. The main use of this pin is to provide the internal +3.3V supply to the USB transceiver cell and the internal 1.5kΩ pull up resistor on USBDP. Up to 50mA can be drawn from this pin to power external logic if required. This pin can also be used to supply the VCCIO pin.
20
VCC
PWR
+3.3V to +5.25V supply to the device core. (see Note 1)
25
AGND
PWR
Device analogue ground supply for internal clock multiplier
Table 3.2 Power and Ground Group
Pin No. Name Type Description
8, 24
NC
NC
No internal connection
19
RESET#
Input
Active low reset pin. This can be used by an external device to reset the FT232R. If not required can be left unconnected, or pulled up to VCC.
26
TEST
Input
Puts the device into IC test mode. Must be tied to GND for normal operation, otherwise the device will appear to fail.
27
OSCI
Input
Input 12MHz Oscillator Cell. Optional – Can be left unconnected for normal operation. (see Note 2)
28
OSCO
Output
Output from 12MHZ Oscillator Cell. Optional – Can be left unconnected for normal operation if internal Oscillator is used. (see Note 2)
Table 3.3 Miscellaneous Signal Group
Pin No. Name Type Description
1
TXD
Output
Transmit Asynchronous Data Output.
2
DTR#
Output
Data Terminal Ready Control Output / Handshake Signal.
3
RTS#
Output
Request to Send Control Output / Handshake Signal.
5
RXD
Input
Receiving Asynchronous Data Input.
6
RI#
Input
Ring Indicator Control Input. When remote wake up is enabled in the internal EEPROM taking RI# low (20ms active low pulse) can be used to resume the PC USB host controller from suspend.
9
DSR#
Input
Data Set Ready Control Input / Handshake Signal.
10
DCD#
Input
Data Carrier Detect Control Input.
11
CTS#
Input
Clear To Send Control Input / Handshake Signal.
12
CBUS4
I/O
Configurable CBUS output only Pin. Function of this pin is configured in the device internal EEPROM. Factory default configuration is SLEEP#. See CBUS Signal Options, Table 3.9.
13
CBUS2
I/O
Configurable CBUS I/O Pin. Function of this pin is configured in the device internal EEPROM. Factory default configuration is TXDEN. See CBUS Signal Options, Table 3.9.
Copyright © 2010 Future Technology Devices International Limited 9
Document No.: FT_000053
FT232R USB UART IC Datasheet Version 2.10
Clearance No.: FTDI# 38
Pin No. Name Type Description
14
CBUS3
I/O
Configurable CBUS I/O Pin. Function of this pin is configured in the device internal EEPROM. Factory default configuration is PWREN#. See CBUS Signal Options, Table 3.9. PWREN# should be used with a 10kΩ resistor pull up.
22
CBUS1
I/O
Configurable CBUS I/O Pin. Function of this pin is configured in the device internal EEPROM. Factory default configuration is RXLED#. See CBUS Signal Options, Table 3.9.
23
CBUS0
I/O
Configurable CBUS I/O Pin. Function of this pin is configured in the device internal EEPROM. Factory default configuration is TXLED#. See CBUS Signal Options, Table 3.9.
Table 3.4 UART Interface and CUSB Group (see note 3)
Notes:
1. The minimum operating voltage VCC must be +4.0V (could use VBUS=+5V) when using the internal clock generator. Operation at +3.3V is possible using an external crystal oscillator.
2. For details on how to use an external crystal, ceramic resonator, or oscillator with the FT232R, please refer Section 7.6
3. When used in Input Mode, the input pins are pulled to VCCIO via internal 200kΩ resistors. These pins can be programmed to gently pull low during USB suspend (PWREN# = “1”) by setting an option in the internal EEPROM.
Copyright © 2010 Future Technology Devices International Limited 10
Document No.: FT_000053
FT232R USB UART IC Datasheet Version 2.10
Clearance No.: FTDI# 38
3.3 QFN-32 Package
Figure 3.2 QFN-32 Package Pin Out and schematic symbol
3.4 QFN-32 Package Signal Description Pin No. Name Type Description
14
USBDP
I/O
USB Data Signal Plus, incorporating internal series resistor and 1.5kΩ pull up resistor to +3.3V.
15
USBDM
I/O
USB Data Signal Minus, incorporating internal series resistor.
Table 3.5 USB Interface Group
Pin No. Name Type Description
1
VCCIO
PWR
+1.8V to +5.25V supply for the UART Interface and CBUS group pins (2, 3, 6,7,8,9,10 11, 21, 22, 30,31,32). In USB bus powered designs connect this pin to 3V3OUT to drive out at +3.3V levels, or connect to VCC to drive out at +5V CMOS level. This pin can also be supplied with an external +1.8V to +2.8V supply in order to drive out at lower levels. It should be noted that in this case this supply should originate from the same source as the supply to VCC. This means that in bus powered designs a regulator which is supplied by the +5V on the USB bus should be used.
4, 17, 20
GND
PWR
Device ground supply pins.
FT232RQ3225241716981YYXX-A1891234567810111213141516171920212223242526272829303132USBDPUSBDM3V3OUTRESET#VCCNCAGNDTESTOSCIOSCOCBUS1CBUS0TXDRTS#RXDDTR#VCCIORI#GNDNCDSR#DCD#CTS#CBUS4CBUS2CBUS3GNDGNDNCNCNCNCIFT232RQAGNDGNDGNDGNDTEST2441720263V3OUTVCCIO116NCRESET#NC231813TXDRXDRTS#CTS#DTR#DSR#DCD#RI#30232831673CBUS0CBUS3CBUS2CBUS122211011191514USBDPUSBDMVCCOSCI27OSCO28CBUS49NC12NC5NC29NC25FTDXXXXXXX
Copyright © 2010 Future Technology Devices International Limited 11
Document No.: FT_000053
FT232R USB UART IC Datasheet Version 2.10
Clearance No.: FTDI# 38
Pin No. Name Type Description
16
3V3OUT
Output
+3.3V output from integrated LDO regulator. This pin should be decoupled to ground using a 100nF capacitor. The purpose of this output is to provide the internal +3.3V supply to the USB transceiver cell and the internal 1.5kΩ pull up resistor on USBDP. Up to 50mA can be drawn from this pin to power external logic if required. This pin can also be used to supply the VCCIO pin.
19
VCC
PWR
+3.3V to +5.25V supply to the device core. (See Note 1).
24
AGND
PWR
Device analogue ground supply for internal clock multiplier.
Table 3.6 Power and Ground Group
Pin No. Name Type Description
5, 12, 13, 23, 25, 29
NC
NC
No internal connection. Do not connect.
18
RESET#
Input
Active low reset. Can be used by an external device to reset the FT232R. If not required can be left unconnected, or pulled up to VCC.
26
TEST
Input
Puts the device into IC test mode. Must be tied to GND for normal operation, otherwise the device will appear to fail.
27
OSCI
Input
Input 12MHz Oscillator Cell. Optional – Can be left unconnected for normal operation. (See Note 2).
28
OSCO
Output
Output from 12MHZ Oscillator Cell. Optional – Can be left unconnected for normal operation if internal Oscillator is used. (See Note 2).
Table 3.7 Miscellaneous Signal Group
Pin No. Name Type Description
30
TXD
Output
Transmit Asynchronous Data Output.
31
DTR#
Output
Data Terminal Ready Control Output / Handshake Signal.
32
RTS#
Output
Request to Send Control Output / Handshake Signal.
2
RXD
Input
Receiving Asynchronous Data Input.
3
RI#
Input
Ring Indicator Control Input. When remote wake up is enabled in the internal EEPROM taking RI# low (20ms active low pulse) can be used to resume the PC USB host controller from suspend.
6
DSR#
Input
Data Set Ready Control Input / Handshake Signal.
7
DCD#
Input
Data Carrier Detect Control Input.
8
CTS#
Input
Clear To Send Control Input / Handshake Signal.
9
CBUS4
I/O
Configurable CBUS output only Pin. Function of this pin is configured in the device internal EEPROM. Factory default configuration is SLEEP#. See CBUS Signal Options, Table 3.9.
10
CBUS2
I/O
Configurable CBUS I/O Pin. Function of this pin is configured in the device internal EEPROM. Factory default configuration is TXDEN. See CBUS Signal Options, Table 3.9.
Copyright © 2010 Future Technology Devices International Limited 12
Document No.: FT_000053
FT232R USB UART IC Datasheet Version 2.10
Clearance No.: FTDI# 38
Pin No. Name Type Description
11
CBUS3
I/O
Configurable CBUS I/O Pin. Function of this pin is configured in the device internal EEPROM. Factory default configuration is PWREN#. See CBUS Signal Options, Table 3.9. PWREN# should be used with a 10kΩ resistor pull up.
21
CBUS1
I/O
Configurable CBUS I/O Pin. Function of this pin is configured in the device internal EEPROM. Factory default configuration is RXLED#. See CBUS Signal Options, Table 3.9.
22
CBUS0
I/O
Configurable CBUS I/O Pin. Function of this pin is configured in the device internal EEPROM. Factory default configuration is TXLED#. See CBUS Signal Options, Table 3.9.
Table 3.8 UART Interface and CBUS Group (see note 3)
Notes:
1. The minimum operating voltage VCC must be +4.0V (could use VBUS=+5V) when using the internal clock generator. Operation at +3.3V is possible using an external crystal oscillator.
2. For details on how to use an external crystal, ceramic resonator, or oscillator with the FT232R, please refer to Section 7.6.
3. When used in Input Mode, the input pins are pulled to VCCIO via internal 200kΩ resistors. These pins can be programmed to gently pull low during USB suspend (PWREN# = “1”) by setting an option in the internal EEPROM.
Copyright © 2010 Future Technology Devices International Limited 13
Document No.: FT_000053
FT232R USB UART IC Datasheet Version 2.10
Clearance No.: FTDI# 38
3.5 CBUS Signal Options
The following options can be configured on the CBUS I/O pins. CBUS signal options are common to both package versions of the FT232R. These options can be configured in the internal EEPROM using the software utility FT_PPROG or MPROG, which can be downloaded from the FTDI Utilities (www.ftdichip.com). The default configuration is described in Section 8. CBUS Signal Option Available On CBUS Pin Description
TXDEN
CBUS0, CBUS1, CBUS2, CBUS3, CBUS4
Enable transmit data for RS485
PWREN#
CBUS0, CBUS1, CBUS2, CBUS3, CBUS4
Output is low after the device has been configured by USB, then high during USB suspend mode. This output can be used to control power to external logic P-Channel logic level MOSFET switch. Enable the interface pull-down option when using the PWREN# in this way.*
TXLED#
CBUS0, CBUS1, CBUS2, CBUS3, CBUS4
Transmit data LED drive: Data from USB Host to FT232R. Pulses low when transmitting data via USB. See Section 7.5 for more details.
RXLED#
CBUS0, CBUS1, CBUS2, CBUS3, CBUS4
Receive data LED drive: Data from FT232R to USB Host. Pulses low when receiving data via USB. See Section 7.5 for more details.
TX&RXLED#
CBUS0, CBUS1, CBUS2, CBUS3, CBUS4
LED drive – pulses low when transmitting or receiving data via USB. See Section 7.5 for more details.
SLEEP#
CBUS0, CBUS1, CBUS2, CBUS3, CBUS4
Goes low during USB suspend mode. Typically used to power down an external TTL to RS232 level converter IC in USB to RS232 converter designs.
CLK48
CBUS0, CBUS1, CBUS2, CBUS3, CBUS4
48MHz ±0.7% Clock output. **
CLK24
CBUS0, CBUS1, CBUS2, CBUS3, CBUS4
24 MHz Clock output.**
CLK12
CBUS0, CBUS1, CBUS2, CBUS3, CBUS4
12 MHz Clock output.**
CLK6
CBUS0, CBUS1, CBUS2, CBUS3, CBUS4
6 MHz ±0.7% Clock output. **
CBitBangI/O
CBUS0, CBUS1, CBUS2, CBUS3
CBUS bit bang mode option. Allows up to 4 of the CBUS pins to be used as general purpose I/O. Configured individually for CBUS0, CBUS1, CBUS2 and CBUS3 in the internal EEPROM. A separate application note, AN232R-01, available from FTDI website (www.ftdichip.com) describes in more detail how to use CBUS bit bang mode.
BitBangWRn
CBUS0, CBUS1, CBUS2, CBUS3
Synchronous and asynchronous bit bang mode WR# strobe output.
BitBangRDn
CBUS0, CBUS1, CBUS2, CBUS3
Synchronous and asynchronous bit bang mode RD# strobe output.
Table 3.9 CBUS Configuration Control
* PWREN# must be used with a 10kΩ resistor pull up.
**When in USB suspend mode the outputs clocks are also suspended.
Copyright © 2010 Future Technology Devices International Limited 14
Document No.: FT_000053
FT232R USB UART IC Datasheet Version 2.10
Clearance No.: FTDI# 38
4 Function Description
The FT232R is a USB to serial UART interface device which simplifies USB to serial designs and reduces external component count by fully integrating an external EEPROM, USB termination resistors and an integrated clock circuit which requires no external crystal, into the device. It has been designed to operate efficiently with a USB host controller by using as little as possible of the total USB bandwidth available.
4.1 Key Features
Functional Integration. Fully integrated EEPROM, USB termination resistors, clock generation, AVCC filtering, POR and LDO regulator.
Configurable CBUS I/O Pin Options. The fully integrated EEPROM allows configuration of the Control Bus (CBUS) functionality, signal inversion and drive strength selection. There are 5 configurable CBUS I/O pins. These configurable options are
1. TXDEN - transmit enable for RS485 designs.
2. PWREN# - Power control for high power, bus powered designs.
3. TXLED# - for pulsing an LED upon transmission of data.
4. RXLED# - for pulsing an LED upon receiving data.
5. TX&RXLED# - which will pulse an LED upon transmission OR reception of data.
6. SLEEP# - indicates that the device going into USB suspend mode.
7. CLK48 / CLK24 / CLK12 / CLK6 - 48MHz, 24MHz, 12MHz, and 6MHz clock output signal options.
The CBUS pins can also be individually configured as GPIO pins, similar to asynchronous bit bang mode. It is possible to use this mode while the UART interface is being used, thus providing up to 4 general purpose I/O pins which are available during normal operation. An application note, AN232R-01, available from FTDI website (www.ftdichip.com) describes this feature.
The CBUS lines can be configured with any one of these output options by setting bits in the internal EEPROM. The device is supplied with the most commonly used pin definitions pre-programmed - see Section 8 for details.
Asynchronous Bit Bang Mode with RD# and WR# Strobes. The FT232R supports FTDI‟s previous chip generation bit-bang mode. In bit-bang mode, the eight UART lines can be switched from the regular interface mode to an 8-bit general purpose I/O port. Data packets can be sent to the device and they will be sequentially sent to the interface at a rate controlled by an internal timer (equivalent to the baud rate pre-scaler). With the FT232R device this mode has been enhanced by outputting the internal RD# and WR# strobes signals which can be used to allow external logic to be clocked by accesses to the bit-bang I/O bus. This option will be described more fully in a separate application note available from FTDI website (www.ftdichip.com).
Synchronous Bit Bang Mode. The FT232R supports synchronous bit bang mode. This mode differs from asynchronous bit bang mode in that the interface pins are only read when the device is written to. This makes it easier for the controlling program to measure the response to an output stimulus as the data returned is synchronous to the output data. An application note, AN232R-01, available from FTDI website (www.ftdichip.com) describes this feature.
FTDIChip-ID™. The FT232R also includes the new FTDIChip-ID™ security dongle feature. This FTDIChip-ID™ feature allows a unique number to be burnt into each device during manufacture. This number cannot be reprogrammed. This number is only readable over USB and forms a basis of a security dongle which can be used to protect any customer application software being copied. This allows the possibility of using the FT232R in a dongle for software licensing. Further to this, a renewable license scheme can be implemented based on the FTDIChip-ID™ number when encrypted with other information. This encrypted number can be stored in the user area of the FT232R internal EEPROM, and can be decrypted, then compared with the protected FTDIChip-ID™ to verify that a license is valid. Web based applications can be used to maintain product licensing this way. An application note, AN232R-02, available from FTDI website (www.ftdichip.com) describes this feature.
The FT232R is capable of operating at a voltage supply between +3.3V and +5V with a nominal operational mode current of 15mA and a nominal USB suspend mode current of 70μA. This allows greater margin for peripheral designs to meet the USB suspend mode current limit of 2.5mA. An integrated level converter within the UART interface allows the FT232R to interface to UART logic running at +1.8V, 2.5V, +3.3V or +5V.
Copyright © 2010 Future Technology Devices International Limited 15
Document No.: FT_000053
FT232R USB UART IC Datasheet Version 2.10
Clearance No.: FTDI# 38
4.2 Functional Block Descriptions
The following paragraphs detail each function within the FT232R. Please refer to the block diagram shown in Figure 2.1
Internal EEPROM. The internal EEPROM in the FT232R is used to store USB Vendor ID (VID), Product ID (PID), device serial number, product description string and various other USB configuration descriptors. The internal EEPROM is also used to configure the CBUS pin functions. The FT232R is supplied with the internal EEPROM pre-programmed as described in Section 8. A user area of the internal EEPROM is available to system designers to allow storing additional data. The internal EEPROM descriptors can be programmed in circuit, over USB without any additional voltage requirement. It can be programmed using the FTDI utility software called MPROG, which can be downloaded from FTDI Utilities on the FTDI website (www.ftdichip.com).
+3.3V LDO Regulator. The +3.3V LDO regulator generates the +3.3V reference voltage for driving the USB transceiver cell output buffers. It requires an external decoupling capacitor to be attached to the 3V3OUT regulator output pin. It also provides +3.3V power to the 1.5kΩ internal pull up resistor on USBDP. The main function of the LDO is to power the USB Transceiver and the Reset Generator Cells rather than to power external logic. However, it can be used to supply external circuitry requiring a +3.3V nominal supply with a maximum current of 50mA.
USB Transceiver. The USB Transceiver Cell provides the USB 1.1 / USB 2.0 full-speed physical interface to the USB cable. The output drivers provide +3.3V level slew rate control signalling, whilst a differential input receiver and two single ended input receivers provide USB data in, Single-Ended-0 (SE0) and USB reset detection conditions respectfully. This function also incorporates the internal USB series termination resistors on the USB data lines and a 1.5kΩ pull up resistor on USBDP.
USB DPLL. The USB DPLL cell locks on to the incoming NRZI USB data and generates recovered clock and data signals for the Serial Interface Engine (SIE) block.
Internal 12MHz Oscillator - The Internal 12MHz Oscillator cell generates a 12MHz reference clock. This provides an input to the x4 Clock Multiplier function. The 12MHz Oscillator is also used as the reference clock for the SIE, USB Protocol Engine and UART FIFO controller blocks.
Clock Multiplier / Divider. The Clock Multiplier / Divider takes the 12MHz input from the Internal Oscillator function and generates the 48MHz, 24MHz, 12MHz and 6MHz reference clock signals. The 48Mz clock reference is used by the USB DPLL and the Baud Rate Generator blocks.
Serial Interface Engine (SIE). The Serial Interface Engine (SIE) block performs the parallel to serial and serial to parallel conversion of the USB data. In accordance with the USB 2.0 specification, it performs bit stuffing/un-stuffing and CRC5/CRC16 generation. It also checks the CRC on the USB data stream.
USB Protocol Engine. The USB Protocol Engine manages the data stream from the device USB control endpoint. It handles the low level USB protocol requests generated by the USB host controller and the commands for controlling the functional parameters of the UART in accordance with the USB 2.0 specification chapter 9.
FIFO RX Buffer (128 bytes). Data sent from the USB host controller to the UART via the USB data OUT endpoint is stored in the FIFO RX (receive) buffer. Data is removed from the buffer to the UART transmit register under control of the UART FIFO controller. (Rx relative to the USB interface).
FIFO TX Buffer (256 bytes). Data from the UART receive register is stored in the TX buffer. The USB host controller removes data from the FIFO TX Buffer by sending a USB request for data from the device data IN endpoint. (Tx relative to the USB interface).
UART FIFO Controller. The UART FIFO controller handles the transfer of data between the FIFO RX and TX buffers and the UART transmit and receive registers.
UART Controller with Programmable Signal Inversion and High Drive. Together with the UART FIFO Controller the UART Controller handles the transfer of data between the FIFO RX and FIFO TX buffers and the UART transmit and receive registers. It performs asynchronous 7 or 8 bit parallel to serial and serial to parallel conversion of the data on the RS232 (or RS422 or RS485) interface.
Control signals supported by UART mode include RTS, CTS, DSR, DTR, DCD and RI. The UART Controller also provides a transmitter enable control signal pin option (TXDEN) to assist with interfacing to RS485 transceivers. RTS/CTS, DSR/DTR and XON / XOFF handshaking options are also supported. Handshaking is handled in hardware to ensure fast response times. The UART interface also supports the RS232 BREAK setting and detection conditions.
Copyright © 2010 Future Technology Devices International Limited 16
Document No.: FT_000053
FT232R USB UART IC Datasheet Version 2.10
Clearance No.: FTDI# 38
Additionally, the UART signals can each be individually inverted and have a configurable high drive strength capability. Both these features are configurable in the EEPROM.
Baud Rate Generator - The Baud Rate Generator provides a 16x clock input to the UART Controller from the 48MHz reference clock. It consists of a 14 bit pre-scaler and 3 register bits which provide fine tuning of the baud rate (used to divide by a number plus a fraction or “sub-integer”). This determines the baud rate of the UART, which is programmable from 183 baud to 3 Mbaud.
The FT232R supports all standard baud rates and non-standard baud rates from 183 Baud up to 3 Mbaud. Achievable non-standard baud rates are calculated as follows -
Baud Rate = 3000000 / (n + x)
where „n‟ can be any integer between 2 and 16,384 ( = 214 ) and „x’ can be a sub-integer of the value 0, 0.125, 0.25, 0.375, 0.5, 0.625, 0.75, or 0.875. When n = 1, x = 0, i.e. baud rate divisors with values between 1 and 2 are not possible.
This gives achievable baud rates in the range 183.1 baud to 3,000,000 baud. When a non-standard baud rate is required simply pass the required baud rate value to the driver as normal, and the FTDI driver will calculate the required divisor, and set the baud rate. See FTDI application note AN232B-05 on the FTDI website (www.ftdichip.com) for more details.
RESET Generator - The integrated Reset Generator Cell provides a reliable power-on reset to the device internal circuitry at power up. The RESET# input pin allows an external device to reset the FT232R.
RESET# can be tied to VCC or left unconnected if not being used.
Copyright © 2010 Future Technology Devices International Limited 17
Document No.: FT_000053
FT232R USB UART IC Datasheet Version 2.10
Clearance No.: FTDI# 38
5 Devices Characteristics and Ratings
5.1 Absolute Maximum Ratings
The absolute maximum ratings for the FT232R devices are as follows. These are in accordance with the Absolute Maximum Rating System (IEC 60134). Exceeding these may cause permanent damage to the device. Parameter Value Unit
Storage Temperature
-65°C to 150°C
Degrees C
Floor Life (Out of Bag) At Factory Ambient
(30°C / 60% Relative Humidity)
168 Hours
(IPC/JEDEC J-STD-033A MSL Level 3 Compliant)*
Hours
Ambient Temperature (Power Applied)
-40°C to 85°C
Degrees C
MTTF FT232RL
11162037
hours
MTTF FT232RQ
4464815
hours
VCC Supply Voltage
-0.5 to +6.00
V
DC Input Voltage – USBDP and USBDM
-0.5 to +3.8
V
DC Input Voltage – High Impedance Bidirectionals
-0.5 to + (VCC +0.5)
V
DC Input Voltage – All Other Inputs
-0.5 to + (VCC +0.5)
V
DC Output Current – Outputs
24
mA
DC Output Current – Low Impedance Bidirectionals
24
mA
Power Dissipation (VCC = 5.25V)
500
mW
Table 5.1 Absolute Maximum Ratings
* If devices are stored out of the packaging beyond this time limit the devices should be baked before use. The devices should be ramped up to a temperature of +125°C and baked for up to 17 hours.
Copyright © 2010 Future Technology Devices International Limited 18
Document No.: FT_000053
FT232R USB UART IC Datasheet Version 2.10
Clearance No.: FTDI# 38
5.2 DC Characteristics
DC Characteristics (Ambient Temperature = -40°C to +85°C) Parameter Description Minimum Typical Maximum Units Conditions
VCC1
VCC Operating Supply Voltage
4.0
---
5.25
V
Using Internal Oscillator
VCC1
VCC Operating Supply Voltage
3.3
---
5.25
V
Using External Crystal
VCC2
VCCIO Operating Supply Voltage
1.8
---
5.25
V
Icc1
Operating Supply Current
---
15
---
mA
Normal Operation
Icc2
Operating Supply Current
50
70
100
μA
USB Suspend
3V3
3.3v regulator output
3.0
3.3
3.6
V
Table 5.2 Operating Voltage and Current
Parameter Description Minimum Typical Maximum Units Conditions
Voh
Output Voltage High
3.2
4.1
4.9
V
I source = 2mA
Vol
Output Voltage Low
0.3
0.4
0.6
V
I sink = 2mA
Vin
Input Switching Threshold
1.0
1.2
1.5
V
**
VHys
Input Switching Hysteresis
20
25
30
mV
**
Table 5.3 UART and CBUS I/O Pin Characteristics (VCCIO = +5.0V, Standard Drive Level)
Parameter Description Minimum Typical Maximum Units Conditions
Voh
Output Voltage High
2.2
2.7
3.2
V
I source = 1mA
Vol
Output Voltage Low
0.3
0.4
0.5
V
I sink = 2mA
Vin
Input Switching Threshold
1.0
1.2
1.5
V
**
VHys
Input Switching Hysteresis
20
25
30
mV
**
Table 5.4 UART and CBUS I/O Pin Characteristics (VCCIO = +3.3V, Standard Drive Level)
Copyright © 2010 Future Technology Devices International Limited 19
Document No.: FT_000053
FT232R USB UART IC Datasheet Version 2.10
Clearance No.: FTDI# 38
Parameter Description Minimum Typical Maximum Units Conditions
Voh
Output Voltage High
2.1
2.6
2.8
V
I source = 1mA
Vol
Output Voltage Low
0.3
0.4
0.5
V
I sink = 2mA
Vin
Input Switching Threshold
1.0
1.2
1.5
V
**
VHys
Input Switching Hysteresis
20
25
30
mV
**
Table 5.5 UART and CBUS I/O Pin Characteristics (VCCIO = +2.8V, Standard Drive Level)
Parameter Description Minimum Typical Maximum Units Conditions
Voh
Output Voltage High
1.32
1.62
1.8
V
I source = 0.2mA
Vol
Output Voltage Low
0.06
0.1
0.18
V
I sink = 0.5mA
Vin
Input Switching Threshold
1.0
1.2
1.5
V
**
VHys
Input Switching Hysteresis
20
25
30
mV
**
Table 5.6 UART and CBUS I/O Pin Characteristics (VCCIO = +1.8V, Standard Drive Level)
Parameter Description Minimum Typical Maximum Units Conditions
Voh
Output Voltage High
3.2
4.1
4.9
V
I source = 6mA
Vol
Output Voltage Low
0.3
0.4
0.6
V
I sink = 6mA
Vin
Input Switching Threshold
1.0
1.2
1.5
V
**
VHys
Input Switching Hysteresis
20
25
30
mV
**
Table 5.7 UART and CBUS I/O Pin Characteristics (VCCIO = +5.0V, High Drive Level)
Parameter Description Minimum Typical Maximum Units Conditions
Voh
Output Voltage High
2.2
2.8
3.2
V
I source = 3mA
Vol
Output Voltage Low
0.3
0.4
0.6
V
I sink = 8mA
Vin
Input Switching Threshold
1.0
1.2
1.5
V
**
VHys
Input Switching Hysteresis
20
25
30
mV
**
Table 5.8 UART and CBUS I/O Pin Characteristics (VCCIO = +3.3V, High Drive Level)
Copyright © 2010 Future Technology Devices International Limited 20
Document No.: FT_000053
FT232R USB UART IC Datasheet Version 2.10
Clearance No.: FTDI# 38
Parameter Description Minimum Typical Maximum Units Conditions
Voh
Output Voltage High
2.1
2.6
2.8
V
I source = 3mA
Vol
Output Voltage Low
0.3
0.4
0.6
V
I sink = 8mA
Vin
Input Switching Threshold
1.0
1.2
1.5
V
**
VHys
Input Switching Hysteresis
20
25
30
mV
**
Table 5.9 UART and CBUS I/O Pin Characteristics (VCCIO = +2.8V, High Drive Level)
Parameter Description Minimum Typical Maximum Units Conditions
Voh
Output Voltage High
1.35
1.67
1.8
V
I source = 0.4mA
Vol
Output Voltage Low
0.12
0.18
0.35
V
I sink = 3mA
Vin
Input Switching Threshold
1.0
1.2
1.5
V
**
VHys
Input Switching Hysteresis
20
25
30
mV
**
Table 5.10 UART and CBUS I/O Pin Characteristics (VCCIO = +1.8V, High Drive Level)
** Only input pins have an internal 200KΩ pull-up resistor to VCCIO
Parameter Description Minimum Typical Maximum Units Conditions
Vin
Input Switching Threshold
1.3
1.6
1.9
V
VHys
Input Switching Hysteresis
50
55
60
mV
Table 5.11 RESET# and TEST Pin Characteristics
Copyright © 2010 Future Technology Devices International Limited 21
Document No.: FT_000053
FT232R USB UART IC Datasheet Version 2.10
Clearance No.: FTDI# 38
Parameter Description Minimum Typical Maximum Units Conditions
UVoh
I/O Pins Static Output (High)
2.8
3.6
V
RI = 1.5kΩ to 3V3OUT (D+) RI = 15KΩ to GND (D-)
UVol
I/O Pins Static Output (Low)
0
0.3
V
RI = 1.5kΩ to 3V3OUT (D+) RI = 15kΩ to GND (D-)
UVse
Single Ended Rx Threshold
0.8
2.0
V
UCom
Differential Common Mode
0.8
2.5
V
UVDif
Differential Input Sensitivity
0.2
V
UDrvZ
Driver Output Impedance
26
29
44
Ohms
See Note 1
Table 5.12 USB I/O Pin (USBDP, USBDM) Characteristics
5.3 EEPROM Reliability Characteristics
The internal 1024 Bit EEPROM has the following reliability characteristics: Parameter Value Unit
Data Retention
10
Years
Read / Write Cycle
10,000
Cycles
Table 5.13 EEPROM Characteristics
5.4 Internal Clock Characteristics
The internal Clock Oscillator has the following characteristics: Parameter Value Unit Minimum Typical Maximum
Frequency of Operation (see Note 1)
11.98
12.00
12.02
MHz
Clock Period
83.19
83.33
83.47
ns
Duty Cycle
45
50
55
%
Table 5.14 Internal Clock Characteristics
Note 1: Equivalent to +/-1667ppm
Copyright © 2010 Future Technology Devices International Limited 22
Document No.: FT_000053
FT232R USB UART IC Datasheet Version 2.10
Clearance No.: FTDI# 38
Parameter Description Minimum Typical Maximum Units Conditions
Voh
Output Voltage High
2.1
2.8
3.2
V
I source = 3mA
Vol
Output Voltage Low
0.3
0.4
0.6
V
I sink = 8mA
Vin
Input Switching Threshold
1.0
1.2
1.5
V
Table 5.15 OSCI, OSCO Pin Characteristics – see Note 1
Note1: When supplied, the FT232R is configured to use its internal clock oscillator. These characteristics only apply when an external oscillator or crystal is used.
Copyright © 2010 Future Technology Devices International Limited 23
Document No.: FT_000053
FT232R USB UART IC Datasheet Version 2.10
Clearance No.: FTDI# 38
6 USB Power Configurations
The following sections illustrate possible USB power configurations for the FT232R. The illustrations have omitted pin numbers for ease of understanding since the pins differ between the FT232RL and FT232RQ package options.
All USB power configurations illustrated apply to both package options for the FT232R device. Please refer to Section 3 for the package option pin-out and signal descriptions.
6.1 USB Bus Powered Configuration
Figure 6.1 Bus Powered Configuration
Figure 6.1 Illustrates the FT232R in a typical USB bus powered design configuration. A USB bus powered device gets its power from the USB bus. Basic rules for USB bus power devices are as follows –
i) On plug-in to USB, the device should draw no more current than 100mA.
ii) In USB Suspend mode the device should draw no more than 2.5mA.
iii) A bus powered high power USB device (one that draws more than 100mA) should use one of the CBUS pins configured as PWREN# and use it to keep the current below 100mA on plug-in and 2.5mA on USB suspend.
iv) A device that consumes more than 100mA cannot be plugged into a USB bus powered hub.
v) No device can draw more than 500mA from the USB bus.
The power descriptors in the internal EEPROM of the FT232R should be programmed to match the current drawn by the device.
A ferrite bead is connected in series with the USB power supply to reduce EMI noise from the FT232R and associated circuitry being radiated down the USB cable to the USB host. The value of the Ferrite Bead depends on the total current drawn by the application. A suitable range of Ferrite Beads is available from Steward (www.steward.com), for example Steward Part # MI0805K400R-10.
Note: If using PWREN# (available using the CBUS) the pin should be pulled to VCCIO using a 10kΩ resistor.
FT232RAGNDGNDGNDGNDTEST100nF3V3OUTVCCIONCRESET#NC+100nF10nFVccTXDRXDRTS#CTS#DTR#DSR#DCD#RI#CBUS0CBUS3CBUS2CBUS1USBDPUSBDMVCC12345OSCIOSCOCBUS4FerriteBead+4.7uFSHIELDGNDGNDGNDGNDVcc
Copyright © 2010 Future Technology Devices International Limited 24
Document No.: FT_000053
FT232R USB UART IC Datasheet Version 2.10
Clearance No.: FTDI# 38
6.2 Self Powered Configuration
Figure 6.2 Self Powered Configuration
Figure 6.2 illustrates the FT232R in a typical USB self powered configuration. A USB self powered device gets its power from its own power supply, VCC, and does not draw current from the USB bus. The basic rules for USB self powered devices are as follows –
i) A self powered device should not force current down the USB bus when the USB host or hub controller is powered down.
ii) A self powered device can use as much current as it needs during normal operation and USB suspend as it has its own power supply.
iii) A self powered device can be used with any USB host, a bus powered USB hub or a self powered USB hub.
The power descriptor in the internal EEPROM of the FT232R should be programmed to a value of zero (self powered).
In order to comply with the first requirement above, the USB bus power (pin 1) is used to control the RESET# pin of the FT232R device. When the USB host or hub is powered up an internal 1.5kΩ resistor on USBDP is pulled up to +3.3V (generated using the 4K7 and 10k resistor network), thus identifying the device as a full speed device to the USB host or hub. When the USB host or hub is powered off, RESET# will be low and the FT232R is held in reset. Since RESET# is low, the internal 1.5kΩ resistor is not pulled up to any power supply (hub or host is powered down), so no current flows down USBDP via the 1.5kΩ pull-up resistor. Failure to do this may cause some USB host or hub controllers to power up erratically.
Figure 6.2 illustrates a self powered design which has a +4V to +5.25V supply.
Note:
1. When the FT232R is in reset, the UART interface I/O pins are tri-stated. Input pins have internal 200kΩ pull-up resistors to VCCIO, so they will gently pull high unless driven by some external logic.
2. When using internal FT232R oscillator the VCC supply voltage range must be +4.0V to 5.25V.
3. When using external oscillator the VCC supply voltage range must be +3.3V to 5.25V
Any design which interfaces to +3.3 V or +1.8V would be having a +3.3V or +1.8V supply to VCCIO.
Copyright © 2010 Future Technology Devices International Limited 25
Document No.: FT_000053
FT232R USB UART IC Datasheet Version 2.10
Clearance No.: FTDI# 38
6.3 USB Bus Powered with Power Switching Configuration
FT232R
GND
GND
100nF
VCC
USBDM
USBDP
VCCIO
NC
RESET#
NC
OSCI
OSCO
3V3OUT
A
G
N
D
G
N
D
G
N
D
G
N
D
T
E
S
T
TXD
RXD
RTS#
CTS#
DTR#
DSR#
DCD#
RI#
CBUS0
CBUS1
CBUS2
CBUS3
CBUS4
1
2
3
4
GND
SHIELD
GND
100nF 4.7uF +
5
10nF +
Ferrite Bead
s d
g
P-Channel Power
MOSFET
PWREN#
1K
Switched 5V Power
To External Logic
Soft Start
Circuit
0.1uF 0.1uF
5V VCC
5V VCC
5V VCC
10K
Figure 6.3 Bus Powered with Power Switching Configuration
A requirement of USB bus powered applications, is when in USB suspend mode, the application draws a
total current of less than 2.5mA. This requirement includes external logic. Some external logic has the
ability to power itself down into a low current state by monitoring the PWREN# signal. For external logic
that cannot power itself down in this way, the FT232R provides a simple but effective method of turning
off power during the USB suspend mode.
Figure 6.3 shows an example of using a discrete P-Channel MOSFET to control the power to external
logic. A suitable device to do this is an International Rectifier (www.irf.com) IRLML6402, or equivalent. It
is recommended that a “soft start” circuit consisting of a 1kΩ series resistor and a 0.1μF capacitor is used
to limit the current surge when the MOSFET turns on. Without the soft start circuit it is possible that the
transient power surge, caused when the MOSFET switches on, will reset the FT232R or the USB host/hub
controller. The soft start circuit example shown in Figure 6.3 powers up with a slew rate of
approximaely12.5V/ms. Thus supply voltage to external logic transitions from GND to +5V in
approximately 400 microseconds.
As an alternative to the MOSFET, a dedicated power switch IC with inbuilt “soft-start” can be used. A
suitable power switch IC for such an application is the Micrel (www.micrel.com) MIC2025-2BM or
equivalent.
With power switching controlled designs the following should be noted:
i) The external logic to which the power is being switched should have its own reset circuitry to
automatically reset the logic when power is re-applied when moving out of suspend mode.
ii) Set the Pull-down on Suspend option in the internal FT232R EEPROM.
iii) One of the CBUS Pins should be configured as PWREN# in the internal FT232R EEPROM, and used
to switch the power supply to the external circuitry. This should be pulled high through a 10 kΩ
resistor.
iv) For USB high-power bus powered applications (one that consumes greater than 100mA, and up
to 500mA of current from the USB bus), the power consumption of the application must be set in
the Max Power field in the internal FT232R EEPROM. A high-power bus powered application uses
the descriptor in the internal FT232R EEPROM to inform the system of its power requirements.
v) PWREN# gets its VCC from VCCIO. For designs using 3V3 logic, ensure VCCIO is not powered
down using the external logic. In this case use the +3V3OUT.
Copyright © 2010 Future Technology Devices International Limited 26
Document No.: FT_000053
FT232R USB UART IC Datasheet Version 2.10
Clearance No.: FTDI# 38
6.4 USB Bus Powered with Selectable External Logic Supply
FT232R
A
G
N
D
G
N
D
G
N
D
G
N
D
T
E
S
T
100nF
3V3OUT
VCCIO
NC
RESET#
NC
10nF
TXD
RXD
RTS#
CTS#
DTR#
DSR#
DCD#
RI#
CBUS0
CBUS3
CBUS2
CBUS1
USBDP
USBDM
1 VCC
2
3
4
5
OSCI
OSCO
CBUS4
Ferrite
Bead
+
SHIELD
GND
GND
GND
3.3V or 5V
Supply to
External Logic
100nF
+ 100nF
Vcc
4.7uF
GND
1
Jumper
SLEEP#
PWREN#
2
3
Vcc
VCCIO
10K
VCCIO
Figure 6.4 USB Bus Powered with +3.3V or +5V External Logic Power Supply
Figure 6.4 illustrates a USB bus power application with selectable external logic supply. The external logic
can be selected between +3.3V and +5V using the jumper switch. This jumper is used to allow the
FT232R to be interfaced with a +3.3V or +5V logic devices. The VCCIO pin is either supplied with +5V
from the USB bus (jumper pins1 and 2 connected), or from the +3.3V output from the FT232R 3V3OUT
pin (jumper pins 2 and 3 connected). The supply to VCCIO is also used to supply external logic.
With bus powered applications, the following should be noted:
i) To comply with the 2.5mA current supply limit during USB suspend mode, PWREN# or
SLEEP# signals should be used to power down external logic in this mode. If this is not
possible, use the configuration shown in Section 6.3.
ii) The maximum current sourced from the USB bus during normal operation should not exceed
100mA, otherwise a bus powered design with power switching (Section 6.3) should be used.
Another possible configuration could use a discrete low dropout (LDO) regulator which is supplied by the
5V on the USB bus to supply between +1.8V and +2.8V to the VCCIO pin and to the external logic. In
this case VCC would be supplied with the +5V from the USB bus and the VCCIO would be supplied from
the output of the LDO regulator. This results in the FT232R I/O pins driving out at between +1.8V and
+2.8V logic levels.
For a USB bus powered application, it is important to consider the following when selecting the regulator:
i) The regulator must be capable of sustaining its output voltage with an input voltage of
+4.35V. An Low Drop Out (LDO) regulator should be selected.
ii) The quiescent current of the regulator must be low enough to meet the total current
requirement of <= 2.5mA during USB suspend mode.
A suitable series of LDO regulators that meets these requirements is the MicroChip/Telcom
(www.microchip.com) TC55 series of devices. These devices can supply up to 250mA current and have a
quiescent current of under 1μA.
Copyright © 2010 Future Technology Devices International Limited 27
Document No.: FT_000053
FT232R USB UART IC Datasheet Version 2.10
Clearance No.: FTDI# 38
7 Application Examples
The following sections illustrate possible applications of the FT232R. The illustrations have omitted pin
numbers for ease of understanding since the pins differ between the FT232RL and FT232RQ package
options.
7.1 USB to RS232 Converter
FT232R
GND
GND
100nF
VCC
USBDM
USBDP
VCCIO
NC
RESET#
NC
OSCI
OSCO
3V3OUT
A
G
N
D
G
N
D
G
N
D
G
N
D
T
E
S
T
TXD
RXD
RTS#
CTS#
DTR#
DSR#
DCD#
RI#
CBUS0
CBUS1
CBUS2
CBUS3
CBUS4
1
2
3
4
GND
SHIELD
GND
100nF 4.7uF +
5
10nF +
Ferrite Bead
VCC
VCC
SLEEP#
GPIO2
GPIO3
TXD
RXD
RTS#
CTS#
DTR#
DSR#
DCD#
RI#
RS232 LEVEL
CONVERTER
TXDATA
RXDATA
RTS
CTS
DTR
DSR
DCD
RI
TXLED#
RXLED#
VCC VCC
270R 270R
GND
RI
DTR
CTS
TXDATA
RTS
RXDATA
DSR
DCD
DB9M
SHIELD 10
5
9
48
3
7
2
6
1
SHDN#
Figure 7.1 Application Example showing USB to RS232 Converter
An example of using the FT232R as a USB to RS232 converter is illustrated in Figure 7.1. In this
application, a TTL to RS232 Level Converter IC is used on the serial UART interface of the FT232R to
convert the TTL levels of the FT232R to RS232 levels. This level shift can be done using the popular “213”
series of TTL to RS232 level converters. These “213” devices typically have 4 transmitters and 5 receivers
in a 28-LD SSOP package and feature an in-built voltage converter to convert the +5V (nominal) VCC to
the +/- 9 volts required by RS232. A useful feature of these devices is the SHDN# pin which can be used
to power down the device to a low quiescent current during USB suspend mode.
A suitable level shifting device is the Sipex SP213EHCA which is capable of RS232 communication at up
to 500k baud. If a lower baud rate is acceptable, then several pin compatible alternatives are available
such as the Sipex SP213ECA, the Maxim MAX213CAI and the Analogue Devices ADM213E, which are all
suitable for communication at up to 115.2k baud. If a higher baud rate is required, the Maxim
MAX3245CAI device is capable of RS232 communication rates up to 1Mbaud. Note that the MAX3245 is
not pin compatible with the 213 series devices and that the SHDN pin on the MAX device is active high
and should be connect to PWREN# pin instead of SLEEP# pin.
In example shown, the CBUS0 and CBUS1 have been configured as TXLED# and RXLED# and are being
used to drive two LEDs.
Copyright © 2010 Future Technology Devices International Limited 28
Document No.: FT_000053
FT232R USB UART IC Datasheet Version 2.10
Clearance No.: FTDI# 38
7.2 USB to RS485 Coverter
FT232R
GND
GND
100nF
VCC
USBDM
USBDP
VCCIO
NC
RESET#
NC
OSCI
OSCO
3V3OUT
A
G
N
D
G
N
D
G
N
D
G
N
D
T
E
S
T
TXD
RXD
RTS#
CTS#
DTR#
DSR#
DCD#
RI#
CBUS0
CBUS1
CBUS2
CBUS3
CBUS4
1
2
3
4
GND
SHIELD
GND
100nF 4.7uF +
5
10nF +
Ferrite Bead
Vcc
Vcc
TXD
RXD
GND
DB9M
SHIELD 10
TXDEN
GPO
PWREN#
GPIO0
GPIO1
VCCIO
10K
RS485 LEVEL
CONVERTER
Vcc
SP481
5
1
2
3
4
Link
120R
7
6
Figure 7.2 Application Example Showing USB to RS485 Converter
An example of using the FT232R as a USB to RS485 converter is shown in Figure 7.2. In this application,
a TTL to RS485 level converter IC is used on the serial UART interface of the FT232R to convert the TTL
levels of the FT232R to RS485 levels.
This example uses the Sipex SP481 device. Equivalent devices are available from Maxim and Analogue
Devices. The SP481 is a RS485 device in a compact 8 pin SOP package. It has separate enables on both
the transmitter and receiver. With RS485, the transmitter is only enabled when a character is being
transmitted from the UART. The TXDEN signal CBUS pin option on the FT232R is provided for exactly this
purpose and so the transmitter enable is wired to CBUS2 which has been configured as TXDEN. Similarly,
CBUS3 has been configured as PWREN#. This signal is used to control the SP481‟s receiver enable. The
receiver enable is active low, so it is wired to the PWREN# pin to disable the receiver when in USB
suspend mode. CBUS2 = TXDEN and CBUS3 = PWREN# are the default device configurations of the
FT232R pins.
RS485 is a multi-drop network; so many devices can communicate with each other over a two wire cable
interface. The RS485 cable requires to be terminated at each end of the cable. A link (which provides the
120Ω termination) allows the cable to be terminated if the SP481 is physically positioned at either end of
the cable.
In this example the data transmitted by the FT232R is also present on the receive path of the SP481.This
is a common feature of RS485 and requires the application software to remove the transmitted data from
the received data stream. With the FT232R it is possible to do this entirely in hardware by modifying the
example shown in Figure 7.2 by logically OR‟ing the FT232R TXDEN and the SP481 receiver output and
connecting the output of the OR gate to the RXD of the FT232R.
Note that the TXDEN is activated 1 bit period before the start bit. TXDEN is deactivated at the same time
as the stop bit. This is not configurable.
Copyright © 2010 Future Technology Devices International Limited 29
Document No.: FT_000053
FT232R USB UART IC Datasheet Version 2.10
Clearance No.: FTDI# 38
7.3 USB to RS422 Converter
FT232R
GND
GND
100nF
VCC
USBDM
USBDP
VCCIO
NC
RESET#
NC
OSCI
OSCO
3V3OUT
A
G
N
D
G
N
D
G
N
D
G
N
D
T
E
S
T
TXD
RXD
RTS#
CTS#
DTR#
DSR#
DCD#
RI#
CBUS0
CBUS1
CBUS2
CBUS3
CBUS4-
1
2
3
4
GND
SHIELD
GND
100nF 4.7uF +
5
10nF +
Ferrite Bead
Vcc
Vcc
PWREN#
RS422 LEVEL
CONVERTER
Vcc
SP491
5
3
4
6 7
TXDM
TXDP
RXDP
RXDM
120R
10
9
11
12
SLEEP#
RS422 LEVEL
CONVERTER
SP491
3
4
6 7
Vcc
Vcc
10K
2
5
120R
11
12
9
10
RTSM
RTSP
CTSP
CTSM
GND
DB9M
SHIELD
TXDM
TXDP
RXDP
RXDM
RTSM
RTSP
CTSP
CTSM
2
Figure 7.3 USB to RS422 Converter Configuration
An example of using the FT232R as a USB to RS422 converter is shown in Figure 7.3. In this application,
two TTL to RS422 Level Converter ICs are used on the serial UART interface of the FT232R to convert the
TTL levels of the FT232R to RS422 levels. There are many suitable level converter devices available. This
example uses Sipex SP491 devices which have enables on both the transmitter and receiver. Since the
SP491 transmitter enable is active high, it is connected to a CBUS pin in SLEEP# configuration. The
SP491 receiver enable is active low and is therefore connected to a CBUS pin PWREN# configuration. This
ensures that when both the SP491 transmitters and receivers are enabled then the device is active, and
when the device is in USB suspend mode, the SP491 transmitters and receivers are disabled. If a similar
application is used, but the design is USB BUS powered, it may be necessary to use a P-Channel logic
level MOSFET (controlled by PWREN#) in the VCC line of the SP491 devices to ensure that the USB
standby current of 2.5mA is met.
The SP491 is specified to transmit and receive data at a rate of up to 5 Mbaud. In this example the
maximum data rate is limited to 3 Mbaud by the FT232R.
Copyright © 2010 Future Technology Devices International Limited 30
Document No.: FT_000053
FT232R USB UART IC Datasheet Version 2.10
Clearance No.: FTDI# 38
7.4 USB to MCU UART Interface
FT232R
GND
GND
100nF
VCC
USBDM
USBDP
VCCIO
NC
RESET#
NC
OSCI
OSCO
3V3OUT
A
G
N
D
G
N
D
G
N
D
G
N
D
T
E
S
T
TXD
RXD
RTS#
CTS#
DTR#
DSR#
DCD#
RI#
CBUS0
CBUS1
CBUS2
CBUS3
CBUS4
1
2
3
4
GND
SHIELD
GND
100nF 4.7uF +
5
10nF +
Ferrite Bead
Vcc
Vcc
PWREN#
Vcc
12MHz
OUT
10K
Microcontroller
CLK_IN
I/O
RTS#
RXD
TXD
CTS#
Vcc
Figure 7.4 USB to MCU UART Interface
An example of using the FT232R as a USB to Microcontroller (MCU) UART interface is shown in Figure
7.4. In this application the FT232R uses TXD and RXD for transmission and reception of data, and RTS# /
CTS# signals for hardware handshaking. Also in this example CBUS0 has been configured as a 12MHz
output to clock the MCU.
Optionally, RI# could be connected to another I/O pin on the MCU and used to wake up the USB host
controller from suspend mode. If the MCU is handling power management functions, then a CBUS pin can
be configured as PWREN# and would also be connected to an I/O pin of the MCU.
Copyright © 2010 Future Technology Devices International Limited 31
Document No.: FT_000053
FT232R USB UART IC Datasheet Version 2.10
Clearance No.: FTDI# 38
7.5 LED Interface
Any of the CBUS I/O pins can be configured to drive an LED. The FT232R has 3 configuration options for
driving LEDs from the CBUS. These are TXLED#, RXLED#, and TX&RXLED#. Refer to Section 3.5 for
configuration options.
FT232R
CBUS[0...4]
CBUS[0...4]
VCCIO
TX
TXLED#
RXLED#
RX
270R 270R
Figure 7.5 Dual LED Configuration
An example of using the FT232R to drive LEDs is shown in Figure 7.5. In this application one of the CBUS
pins is used to indicate transmission of data (TXLED#) and another is used to indicate receiving data
(RXLED#). When data is being transmitted or received the respective pins will drive from tri-state to low
in order to provide indication on the LEDs of data transfer. A digital one-shot is used so that even a small
percentage of data transfer is visible to the end user.
FT232R
CBUS[0...4]
TX&RXLED#
270R
VCCIO
LED
Figure 7.6 Single LED Configuration
Another example of using the FT232R to drive LEDs is shown in Figure 7.6. In this example one of the
CBUS pins is used to indicate when data is being transmitted or received by the device (TX&RXLED). In
this configuration the FT232R will drive only a single LED.
Copyright © 2010 Future Technology Devices International Limited 32
Document No.: FT_000053
FT232R USB UART IC Datasheet Version 2.10
Clearance No.: FTDI# 38
7.6 Using the External Oscillator
The FT232R defaults to operating using its own internal oscillator. This requires that the device is powered with VCC(min)=+4.0V. This supply voltage can be taken from the USB VBUS. Applications which require using an external oscillator, VCC= +3.3V, must do so in the following order:
1. When device powered for the very first time, it must have VCC > +4.0V. This supply is available from the USB VBUS supply = +5.0V.
2. The EEPROM must then be programmed to enable external oscillator. This EEPROM modification cannot be done using the FTDI programming utility, MPROG. The EEPROM can only be re-configured from a custom application. Please refer to the following applications note on how to do this:
http://www.ftdichip.com/Documents/AppNotes/AN_100_Using_The_FT232_245R_With_External_Osc(FT_000067).pdf
3. The FT232R can then be powered from VCC=+3.3V and an external oscillator. This can be done using a link to switch the VCC supply.
The FT232R will fail to operate when the internal oscillator has been disabled, but no external oscillator has been connected.
Copyright © 2010 Future Technology Devices International Limited 33
Document No.: FT_000053
FT232R USB UART IC Datasheet Version 2.10
Clearance No.: FTDI# 38
8 Internal EEPROM Configuration
Following a power-on reset or a USB reset the FT232R will scan its internal EEPROM and read the USB configuration descriptors stored there. The default factory programmed values of the internal EEPROM are shown in Table 8.1.
Parameter Value Notes
USB Vendor ID (VID)
0403h
FTDI default VID (hex)
USB Product UD (PID)
6001h
FTDI default PID (hex)
Serial Number Enabled?
Yes
Serial Number
See Note
A unique serial number is generated and programmed into the EEPROM during device final test.
Pull down I/O Pins in USB Suspend
Disabled
Enabling this option will make the device pull down on the UART interface lines when in USB suspend mode (PWREN# is high).
Manufacturer Name
FTDI
Product Description
FT232R USB UART
Max Bus Power Current
90mA
Power Source
Bus Powered
Device Type
FT232R
USB Version
0200
Returns USB 2.0 device description to the host.
Note: The device is a USB 2.0 Full Speed device (12Mb/s) as opposed to a USB 2.0 High Speed device (480Mb/s).
Remote Wake Up
Enabled
Taking RI# low will wake up the USB host controller from suspend in approximately 20 ms.
High Current I/Os
Disabled
Enables the high drive level on the UART and CBUS I/O pins.
Load VCP Driver
Enabled
Makes the device load the VCP driver interface for the device.
CBUS0
TXLED#
Default configuration of CBUS0 – Transmit LED drive.
CBUS1
RXLED#
Default configuration of CBUS1 – Receive LED drive.
CBUS2
TXDEN
Default configuration of CBUS2 – Transmit data enable for RS485
CBUS3
PWREN#
Default configuration of CBUS3 – Power enable. Low after USB enumeration, high during USB suspend mode.
Copyright © 2010 Future Technology Devices International Limited 34
Document No.: FT_000053
FT232R USB UART IC Datasheet Version 2.10
Clearance No.: FTDI# 38
Parameter Value Notes
CBUS4
SLEEP#
Default configuration of CBUS4 – Low during USB suspend mode.
Invert TXD
Disabled
Signal on this pin becomes TXD# if enable.
Invert RXD
Disabled
Signal on this pin becomes RXD# if enable.
Invert RTS#
Disabled
Signal on this pin becomes RTS if enable.
Invert CTS#
Disabled
Signal on this pin becomes CTS if enable.
Invert DTR#
Disabled
Signal on this pin becomes DTR if enable.
Invert DSR#
Disabled
Signal on this pin becomes DSR if enable.
Invert DCD#
Disabled
Signal on this pin becomes DCD if enable.
Invert RI#
Disabled
Signal on this pin becomes RI if enable.
Table 8.1 Default Internal EEPROM Configuration
The internal EEPROM in the FT232R can be programmed over USB using the FTDI utility program MPROG. MPROG can be downloaded from FTDI Utilities on the FTDI website (www.ftdichip.com). Version 2.8a or later is required for the FT232R chip. Users who do not have their own USB Vendor ID but who would like to use a unique Product ID in their design can apply to FTDI for a free block of unique PIDs. Contact FTDI support for this service.
Copyright © 2010 Future Technology Devices International Limited 35
Document No.: FT_000053
FT232R USB UART IC Datasheet Version 2.10
Clearance No.: FTDI# 38
9 Package Parameters
The FT232R is available in two different packages. The FT232RL is the SSOP-28 option and the FT232RQ is the QFN-32 package option. The solder reflow profile for both packages is described in Section 9.5.
9.1 SSOP-28 Package Dimensions
Figure 9.1 SSOP-28 Package Dimensions
The FT232RL is supplied in a RoHS compliant 28 pin SSOP package. The package is lead (Pb) free and uses a „green‟ compound. The package is fully compliant with European Union directive 2002/95/EC.
This package is nominally 5.30mm x 10.20mm body (7.80mm x 10.20mm including pins). The pins are on a 0.65 mm pitch. The above mechanical drawing shows the SSOP-28 package.
All dimensions are in millimetres.
The date code format is YYXX where XX = 2 digit week number, YY = 2 digit year number. This is followed by the revision number.
The code XXXXXXXXXXXX is the manufacturing LOT code. This only applies to devices manufactured after April 2009.
12° Typ0° - 8°0.25 0.75 +/-0.200.092.00 Max1.75+/- 0.100.05 Min1.25 +/-0.12FT232RLYYXX-A1141528FTDI5.30 +/-0.307.80 +/-0.40 10.20 +/-0.301.02 Typ.0.30 +/-0.0120.65 +/-0.026XXXXXXXXXXXX
Copyright © 2010 Future Technology Devices International Limited 36
Document No.: FT_000053
FT232R USB UART IC Datasheet Version 2.10
Clearance No.: FTDI# 38
9.2 QFN-32 Package Dimensions
Figure 9.2 QFN-32 Package Dimensions
The FT232RQ is supplied in a RoHS compliant leadless QFN-32 package. The package is lead ( Pb ) free, and uses a „green‟ compound. The package is fully compliant with European Union directive 2002/95/EC.
This package is nominally 5.00mm x 5.00mm. The solder pads are on a 0.50mm pitch. The above mechanical drawing shows the QFN-32 package. All dimensions are in millimetres.
The centre pad on the base of the FT232RQ is not internally connected, and can be left unconnected, or connected to ground (recommended).
The date code format is YYXX where XX = 2 digit week number, YY = 2 digit year number.
The code XXXXXXX is the manufacturing LOT code. This only applies to devices manufactured after April 2009.
Indicates Pin #1 (Laser Marked)FT232RQ3225241716981YYXX-A5.000 +/-0.0755.000 +/-0.0753.200 +/-0.1003.200 +/-0.1000.5000.250 +/-0.0500.500 +/-0.0500.150 MaxPin #1 ID0.900 +/-0.1000.200 0.0502134567823242221201918172526272829303132161514131211109Note: The pin #1 ID is connected internally to the device’s central heat sink area . It is recommended to ground the central heat sink area of the device. 0.200 MinDimensions in mm.Central Heat Sink AreaFTDIXXXXXXX
Copyright © 2010 Future Technology Devices International Limited 37
Document No.: FT_000053
FT232R USB UART IC Datasheet Version 2.10
Clearance No.: FTDI# 38
9.3 QFN-32 Package Typical Pad Layout
Figure 9.3 Typical Pad Layout for QFN-32 Package
9.4 QFN-32 Package Typical Solder Paste Diagram
2.5 +/- 0.0375
2.5 +/- 0.0375
Figure 9.4 Typical Solder Paste Diagram for QFN-32 Package
1
17
25
0.500
0.30
0.200 Min
0.500
+/-0.050
0.150 Max
0.20
0.100
3.200 +/-0.100
3.200 +/-0.100
2.50
2.50
Optional GND
Connection
Optional GND
Connection
9
Copyright © 2010 Future Technology Devices International Limited 38
Document No.: FT_000053
FT232R USB UART IC Datasheet Version 2.10
Clearance No.: FTDI# 38
9.5 Solder Reflow Profile
The FT232R is supplied in Pb free 28 LD SSOP and QFN-32 packages. The recommended solder reflow profile for both package options is shown in Figure 9.5.
Figure 9.5 FT232R Solder Reflow Profile
The recommended values for the solder reflow profile are detailed in Table 9.1. Values are shown for both a completely Pb free solder process (i.e. the FT232R is used with Pb free solder), and for a non-Pb free solder process (i.e. the FT232R is used with non-Pb free solder). Profile Feature Pb Free Solder Process Non-Pb Free Solder Process
Average Ramp Up Rate (Ts to Tp)
3°C / second Max.
3°C / Second Max.
Preheat - Temperature Min (Ts Min.) - Temperature Max (Ts Max.) - Time (ts Min to ts Max)
150°C 200°C 60 to 120 seconds
100°C
150°C
60 to 120 seconds
Time Maintained Above Critical Temperature TL: - Temperature (TL) - Time (tL)
217°C 60 to 150 seconds
183°C 60 to 150 seconds
Peak Temperature (Tp)
260°C
240°C
Time within 5°C of actual Peak Temperature (tp)
20 to 40 seconds
20 to 40 seconds
Ramp Down Rate
6°C / second Max.
6°C / second Max.
Time for T= 25°C to Peak Temperature, Tp
8 minutes Max.
6 minutes Max.
Table 9.1 Reflow Profile Parameter Values
Critical Zone: whenT is in the rangeT to TTemperature, T
(
Degrees C)Time, t (seconds)25PT = 25º C to TtpTpTLtPreheatStLRamp UpLpRampDownT MaxST MinS
Copyright © 2010 Future Technology Devices International Limited 39
Document No.: FT_000053
FT232R USB UART IC Datasheet Version 2.10
Clearance No.: FTDI# 38
10 Contact Information
Head Office – Glasgow, UK
Future Technology Devices International Limited
Unit 1, 2 Seaward Place
Centurion Business Park
Glasgow, G41 1HH
United Kingdom
Tel: +44 (0) 141 429 2777
Fax: +44 (0) 141 429 2758
E-mail (Sales) sales1@ftdichip.com
E-mail (Support) support1@ftdichip.com
E-mail (General Enquiries) admin1@ftdichip.com
Web Site URL http://www.ftdichip.com
Web Shop URL http://www.ftdichip.com
Branch Office – Taipei, Taiwan
Future Technology Devices International Limited (Taiwan)
2F, No 516, Sec. 1 NeiHu Road
Taipei 114
Taiwan, R.O.C.
Tel: +886 (0) 2 8791 3570
Fax: +886 (0) 2 8791 3576
E-mail (Sales) tw.sales1@ftdichip.com
E-mail (Support) tw.support1@ftdichip.com
E-mail (General Enquiries) tw.admin1@ftdichip.com
Web Site URL http://www.ftdichip.com
Branch Office – Hillsboro, Oregon, USA
Future Technology Devices International Limited (USA)
7235 NW Evergreen Parkway, Suite 600
Hillsboro, OR 97123-5803
USA
Tel: +1 (503) 547 0988
Fax: +1 (503) 547 0987
E-Mail (Sales) us.sales@ftdichip.com
E-Mail (Support) us.admin@ftdichip.com
Web Site URL http://www.ftdichip.com
Branch Office – Shanghai, China
Future Technology Devices International Limited (China)
Room 408, 317 Xianxia Road,
ChangNing District,
ShangHai, China
Tel: +86 (21) 62351596 Fax: +86(21) 62351595
E-Mail (Sales): cn.sales@ftdichip.com
E-Mail (Support): cn.support@ftdichip.com
E-Mail (General Enquiries): cn.admin1@ftdichip.com
Web Site URL: http://www.ftdichip.com
Distributor and Sales Representatives
Please visit the Sales Network page of the FTDI Web site for the contact details of our distributor(s) and sales representative(s) in your country.
Copyright © 2010 Future Technology Devices International Limited 40
Document No.: FT_000053
FT232R USB UART IC Datasheet Version 2.10
Clearance No.: FTDI# 38
Appendix A – References
Useful Application Notes
http://www.ftdichip.com/Documents/AppNotes/AN232R-01_FT232RBitBangModes.pdf
http://www.ftdichip.com/Documents/AppNotes/AN_107_AdvancedDriverOptions_AN_000073.pdf
http://www.ftdichip.com/Documents/AppNotes/AN232R-02_FT232RChipID.pdf
http://www.ftdichip.com/Documents/AppNotes/AN_121_FTDI_Device_EEPROM_User_Area_Usage.pdf
http://www.ftdichip.com/Documents/AppNotes/AN_120_Aliasing_VCP_Baud_Rates.pdf
http://www.ftdichip.com/Documents/AppNotes/AN_100_Using_The_FT232_245R_With_External_Osc(FT_000067).pdf
http://www.ftdichip.com/Resources/Utilities/AN_126_User_Guide_For_FT232_Factory%20test%20utility.pdf
http://www.ftdichip.com/Documents/AppNotes/AN232B-05_BaudRates.pdf
http://www.ftdichip.com/Documents/InstallGuides.htm
Copyright © 2010 Future Technology Devices International Limited 41
Document No.: FT_000053
FT232R USB UART IC Datasheet Version 2.10
Clearance No.: FTDI# 38
Appendix B - List of Figures and Tables
List of Figures
Figure 2.1 FT232R Block Diagram ................................................................................................... 4
Figure 3.1 SSOP Package Pin Out and Schematic Symbol .......................................................... 7
Figure 3.2 QFN-32 Package Pin Out and schematic symbol .............................................................. 10
Figure 6.1 Bus Powered Configuration ........................................................................................... 23
Figure 6.2 Self Powered Configuration ........................................................................................... 24
Figure 6.4 USB Bus Powered with +3.3V or +5V External Logic Power Supply .................................... 26
Figure 7.1 Application Example showing USB to RS232 Converter ..................................................... 27
Figure 7.2 Application Example Showing USB to RS485 Converter .................................................... 28
Figure 7.3 USB to RS422 Converter Configuration ........................................................................... 29
Figure 7.4 USB to MCU UART Interface .......................................................................................... 30
Figure 7.5 Dual LED Configuration ................................................................................................ 31
Figure 7.6 Single LED Configuration .............................................................................................. 31
Figure 9.1 SSOP-28 Package Dimensions ....................................................................................... 35
Figure 9.2 QFN-32 Package Dimensions ......................................................................................... 36
Figure 9.3 Typical Pad Layout for QFN-32 Package .......................................................................... 37
Figure 9.4 Typical Solder Paste Diagram for QFN-32 Package ........................................................... 37
Figure 9.5 FT232R Solder Reflow Profile ........................................................................................ 38
List of Tables
Table 3.1 USB Interface Group ....................................................................................................... 7
Table 3.2 Power and Ground Group ................................................................................................. 8
Table 3.3 Miscellaneous Signal Group .............................................................................................. 8
Table 3.4 UART Interface and CUSB Group (see note 3) .................................................................... 9
Table 3.5 USB Interface Group ..................................................................................................... 10
Table 3.6 Power and Ground Group ............................................................................................... 11
Table 3.7 Miscellaneous Signal Group ............................................................................................ 11
Table 3.8 UART Interface and CBUS Group (see note 3) .................................................................. 12
Table 3.9 CBUS Configuration Control ........................................................................................... 13
Table 5.1 Absolute Maximum Ratings ............................................................................................ 17
Table 5.2 Operating Voltage and Current ....................................................................................... 18
Table 5.3 UART and CBUS I/O Pin Characteristics (VCCIO = +5.0V, Standard Drive Level) .................. 18
Table 5.4 UART and CBUS I/O Pin Characteristics (VCCIO = +3.3V, Standard Drive Level) .................. 18
Table 5.5 UART and CBUS I/O Pin Characteristics (VCCIO = +2.8V, Standard Drive Level) .................. 19
Table 5.6 UART and CBUS I/O Pin Characteristics (VCCIO = +1.8V, Standard Drive Level) .................. 19
Table 5.7 UART and CBUS I/O Pin Characteristics (VCCIO = +5.0V, High Drive Level) ......................... 19
Table 5.8 UART and CBUS I/O Pin Characteristics (VCCIO = +3.3V, High Drive Level) ......................... 19
Table 5.9 UART and CBUS I/O Pin Characteristics (VCCIO = +2.8V, High Drive Level) ......................... 20
Table 5.10 UART and CBUS I/O Pin Characteristics (VCCIO = +1.8V, High Drive Level) ....................... 20
Copyright © 2010 Future Technology Devices International Limited 42
Document No.: FT_000053
FT232R USB UART IC Datasheet Version 2.10
Clearance No.: FTDI# 38
Table 5.11 RESET# and TEST Pin Characteristics ............................................................................ 20
Table 5.12 USB I/O Pin (USBDP, USBDM) Characteristics ................................................................. 21
Table 5.13 EEPROM Characteristics ............................................................................................... 21
Table 5.14 Internal Clock Characteristics ....................................................................................... 21
Table 5.15 OSCI, OSCO Pin Characteristics – see Note 1 ................................................................. 22
Table 8.1 Default Internal EEPROM Configuration ............................................................................ 34
Table 9.1 Reflow Profile Parameter Values ..................................................................................... 38
Copyright © 2010 Future Technology Devices International Limited 43
Document No.: FT_000053
FT232R USB UART IC Datasheet Version 2.10
Clearance No.: FTDI# 38
Appendix C - Revision History
Document Title: USB UART IC FT232R
Document Reference No.: FT_000053
Clearance No.: FTDI# 38
Product Page: http://www.ftdichip.com/FTProducts.htm
Document Feedback: Send Feedback
Version 0.90 Initial Datasheet Created August 2005
Version 0.96 Revised Pre-release datasheet October 2005
Version 1.00 Full datasheet released December 2005
Version 1.02 Minor revisions to datasheet December 2005
Version 1.03 Manufacturer ID added to default EEPROM configuration; Buffer sizes added January 2006
Version 1.04 QFN-32 Pad layout and solder paste diagrams added January 2006
Version 2.00 Reformatted, updated package info, added notes for 3.3V operation; June 2008
Part numbers, TID; added UART and CBUS characteristics for +1.8V;
Corrected RESET#; Added MTTF data;
Corrected the input switching threshold and input hysteresis values for VCCIO=5V
Version 2.01 Corrected pin-out number in table3.2 for GND pin18.
Improved graphics on some Figures.
Add packing details. Changed USB suspend current spec from 500uA to 2.5mA
Corrected Figure 9.2 QFN dimensions. August 2008
Version 2.02 Corrected Tape and Reel quantities.
Added comment “PWREN# should be used with a 10kΩ resistor pull up”.
Replaced TXDEN# with TXDEN since it is active high in various places.
Added lot number to the device markings.
Added 3V3 regulator output tolerance.
Clarified VCC operation and added section headed “Using an external Oscillator”
Updated company contact information. April 2009
Version 2.03 Corrected the RX/TX buffer definitions to be relative to the USB interface June 2009
Version 2.04 Additional dimensions added to QFN solder profile June 2009
Version 2.05 Modified package dimensions to 5.0 x 5.0 +/-0.075mm. December 2009 and Solder paste diagram to 2.50 x 2.50 +/-0.0375mm Added Windows 7 32, 64 bit driver support Added FT_PROG utility references Added Appendix A-references.Figure 2.1 updated. Updated USB-IF TID for Rev B
Version 2.06 Updated section 6.2, Figure 6.2 and the note, May 2010
Updated section 5.3, Table 5.13, EEPROM data retention time
Version 2.07 Added USB Certification Logos July 2010
Version 2.08 Updated USB-IF TID for Rev C April 2011
Version 2.09 Corrected Rev C TID number April 2011
Version 2.10 Table 3.9, added clock output frequency within ±0.7% March 2012
Edited Table 3.9, TXLED# and TXLED# Description
Added feedback links
LM78XX / LM78XXA — 3-Terminal 1 A Positive Voltage Regulator
© 2006 Fairchild Semiconductor Corporation www.fairchildsemi.com
LM78XX / LM78XXA Rev. 1.3.0 1
August 2013
LM78XX / LM78XXA
3-Terminal 1 A Positive Voltage Regulator
Features
• Output Current up to 1 A
• Output Voltages: 5, 6, 8, 9, 10, 12, 15, 18, 24 V
• Thermal Overload Protection
• Short-Circuit Protection
• Output Transistor Safe Operating Area Protection
Ordering Information(1)
Note:
1. Above output voltage tolerance is available at 25°C.
Product Number Output Voltage
Tolerance Package Operating
Temperature Packing Method
LM7805CT
±4%
TO-220
(Single Gauge)
-40°C to +125°C
Rail
LM7806CT
LM7808CT
LM7809CT
LM7810CT
LM7812CT
LM7815CT
LM7818CT
LM7824CT
LM7805ACT
±2% 0°C to +125°C
LM7809ACT
LM7810ACT
LM7812ACT
LM7815ACT
Description
The LM78XX series of three-terminal positive regulators
is available in the TO-220 package and with several fixed
output voltages, making them useful in a wide range of
applications. Each type employs internal current limiting,
thermal shut-down, and safe operating area protection. If
adequate heat sinking is provided, they can deliver over
1 A output current. Although designed primarily as fixedvoltage
regulators, these devices can be used with external
components for adjustable voltages and currents.
1
1. Input
2. GND
3. Output
GND
TO-220 (Single Gauge)
LM78XX / LM78XXA — 3-Terminal 1 A Positive Voltage Regulator
© 2006 Fairchild Semiconductor Corporation www.fairchildsemi.com
LM78XX / LM78XXA Rev. 1.3.0 2
Block Diagram
Figure 1. Block Diagram
Absolute Maximum Ratings
Stresses exceeding the absolute maximum ratings may damage the device. The device may not function or be operable
above the recommended operating conditions and stressing the parts to these levels is not recommended. In addition,
extended exposure to stresses above the recommended operating conditions may affect device reliability. The
absolute maximum ratings are stress ratings only. Values are at TA = 25°C unless otherwise noted.
Symbol Parameter Value Unit
VI Input Voltage
VO = 5 V to 18 V 35
V
VO = 24 V 40
RθJC Thermal Resistance, Junction-Case (TO-220) 5 °C/W
RθJA Thermal Resistance, Junction-Air (TO-220) 65 °C/W
TOPR Operating Temperature Range
LM78xx -40 to +125
°C
LM78xxA 0 to +125
TSTG Storage Temperature Range - 65 to +150 °C
Starting
Circuit
Input
1
Reference
Voltage
Current
Generator
SOA
Protection
Thermal
Protection
Series Pass
Element
Error
Amplifier
Output
3
GND
2
LM78XX / LM78XXA — 3-Terminal 1 A Positive Voltage Regulator
© 2006 Fairchild Semiconductor Corporation www.fairchildsemi.com
LM78XX / LM78XXA Rev. 1.3.0 3
Electrical Characteristics (LM7805)
Refer to the test circuit, -40°C < TJ < 125°C, IO = 500 mA, VI = 10 V, CI = 0.1 μF, unless otherwise specified.
Notes:
2. Load and line regulation are specified at constant junction temperature. Changes in VO due to heating effects
must be taken into account separately. Pulse testing with low duty is used.
3. These parameters, although guaranteed, are not 100% tested in production.
Symbol Parameter Conditions Min. Typ. Max. Unit
VO Output Voltage
TJ = +25°C 4.80 5.00 5.20
IO = 5 mA to 1 A, PO ≤ 15 W, V
VI = 7 V to 20 V 4.75 5.00 5.25
Regline Line Regulation(2) TJ = +25°C
VI = 7 V to 25 V 4.0 100.0
mV
VI = 8 V to 12 V 1.6 50.0
Regload Load Regulation(2) TJ = +25°C
IO = 5 mA to 1.5 A 9.0 100.0
mV
IO = 250 mA to 750 mA 4.0 50.0
IQ Quiescent Current TJ =+25°C 5.0 8.0 mA
ΔIQ
Quiescent Current
Change
IO = 5 mA to 1 A 0.03 0.50
mA
VI = 7 V to 25 V 0.30 1.30
ΔVO/ΔT Output Voltage Drift(3) IO = 5 mA -0.8 mV/°C
VN Output Noise Voltage f = 10 Hz to 100 kHz, TA = +25°C 42.0 μV/VO
RR Ripple Rejection(3) f = 120 Hz, VI = 8 V to 18 V 62.0 73.0 dB
VDROP Dropout Voltage TJ = +25°C, IO = 1 A 2.0 V
RO Output Resistance(3) f = 1 kHz 15.0 mΩ
ISC Short-Circuit Current TJ = +25°C, VI = 35 V 230 mA
IPK Peak Current(3) TJ = +25°C 2.2 A
LM78XX / LM78XXA — 3-Terminal 1 A Positive Voltage Regulator
© 2006 Fairchild Semiconductor Corporation www.fairchildsemi.com
LM78XX / LM78XXA Rev. 1.3.0 4
Electrical Characteristics (LM7806)
Refer to the test circuit, -40°C < TJ < 125°C, IO = 500 mA, VI = 11 V, CI = 0.33 μF,CO = 0.1 μF, unless otherwise
specified.
Notes:
4. Load and line regulation are specified at constant junction temperature. Changes in VO due to heating effects must
be taken into account separately. Pulse testing with low duty is used.
5. These parameters, although guaranteed, are not 100% tested in production.
Symbol Parameter Conditions Min. Typ. Max. Unit
VO Output Voltage
TJ = +25°C 5.75 6.00 6.25
IO = 5 mA to 1 A, PO ≤ 15 W, V
VI = 8.0 V to 21 V 5.70 6.00 6.30
Regline Line Regulation(4) TJ = +25°C
VI = 8 V to 25 V 5.0 120
mV
VI = 9 V to 13 V 1.5 60.0
Regload Load Regulation(4) TJ = +25°C
IO = 5 mA to 1.5 A 9.0 120.0
mV
IO = 250 mA to 750 mA 3.0 60.0
IQ Quiescent Current TJ =+25°C 5.0 8.0 mA
ΔIQ
Quiescent Current
Change
IO = 5 mA to 1 A 0.5
mA
VI = 8 V to 25 V 1.3
ΔVO/ΔT Output Voltage Drift(5) IO = 5 mA -0.8 mV/°C
VN Output Noise Voltage f = 10 Hz to 100 kHz, TA = +25°C 45.0 μV/VO
RR Ripple Rejection(5) f = 120 Hz, VI = 8 V to 18 V 62.0 73.0 dB
VDROP Dropout Voltage TJ = +25°C, IO = 1 A 2.0 V
RO Output Resistance(5) f = 1 kHz 19.0 mΩ
ISC Short-Circuit Current TJ = +25°C, VI = 35 V 250 mA
IPK Peak Current(5) TJ = +25°C 2.2 A
LM78XX / LM78XXA — 3-Terminal 1 A Positive Voltage Regulator
© 2006 Fairchild Semiconductor Corporation www.fairchildsemi.com
LM78XX / LM78XXA Rev. 1.3.0 5
Electrical Characteristics (LM7808)
Refer to the test circuit, -40°C < TJ < 125°C, IO = 500 mA, VI = 14 V, CI = 0.33 μF, CO = 0.1 μF, unless otherwise
specified.
Notes:
6. Load and line regulation are specified at constant junction temperature. Changes in VO due to heating effects must
be taken into account separately. Pulse testing with low duty is used.
7. These parameters, although guaranteed, are not 100% tested in production.
Symbol Parameter Conditions Min. Typ. Max. Unit
VO Output Voltage
TJ = +25°C 7.7 8.0 8.3
IO = 5 mA to 1 A, PO ≤ 15 W, V
VI = 10.5 V to 23 V 7.6 8.0 8.4
Regline Line Regulation(6) TJ = +25°C
VI = 10.5 V to 25 V 5.0 160.0
mV
VI = 11.5 V to 17 V 2.0 80.0
Regload Load Regulation(6) TJ = +25°C
IO = 5 mA to 1.5 A 10.0 160.0
mV
IO = 250 mA to 750 mA 5.0 80.0
IQ Quiescent Current TJ =+25°C 5.0 8.0 mA
ΔIQ
Quiescent Current
Change
IO = 5 mA to 1 A 0.05 0.50
mA
VI = 10.5 V to 25 V 0.5 1.0
ΔVO/ΔT Output Voltage Drift(7) IO = 5 mA -0.8 mV/°C
VN Output Noise Voltage f = 10 Hz to 100 kHz, TA = +25°C 52.0 μV/VO
RR Ripple Rejection(7) f = 120 Hz, VI = 11.5 V to 21.5 V 56.0 73.0 dB
VDROP Dropout Voltage IO = 1 A, TJ = +25°C 2.0 V
RO Output Resistance(7) f = 1 kHz 17.0 mΩ
ISC Short-Circuit Current VI = 35 V, TJ = +25°C 230 mA
IPK Peak Current(7) TJ = +25°C 2.2 A
LM78XX / LM78XXA — 3-Terminal 1 A Positive Voltage Regulator
© 2006 Fairchild Semiconductor Corporation www.fairchildsemi.com
LM78XX / LM78XXA Rev. 1.3.0 6
Electrical Characteristics (LM7809)
Refer to the test circuit, -40°C < TJ < 125°C, IO = 500 mA, VI = 15 V, CI = 0.33 μF,CO = 0.1 μF, unless otherwise
specified.
Notes:
8. Load and line regulation are specified at constant junction temperature. Changes in VO due to heating effects must
be taken into account separately. Pulse testing with low duty is used.
9. These parameters, although guaranteed, are not 100% tested in production.
Symbol Parameter Conditions Min. Typ. Max. Unit
VO Output Voltage
TJ = +25°C 8.65 9.00 9.35
IO = 5 mA to 1 A, PO ≤ 15 W, V
VI = 11.5 V to 24 V 8.60 9.00 9.40
Regline Line Regulation(8) TJ = +25°C
VI = 11.5 V to 25 V 6.0 180.0
mV
VI = 12 V to 17 V 2.0 90.0
Regload Load Regulation(8) TJ = +25°C
IO = 5 mA to 1.5 A 12.0 180.0
mV
IO = 250 mA to 750 mA 4.0 90.0
IQ Quiescent Current TJ =+25°C 5.0 8.0 mA
ΔIQ
Quiescent Current
Change
IO = 5 mA to 1 A 0.5
mA
VI = 11.5 V to 26 V 1.3
ΔVO/ΔT Output Voltage Drift(9) IO = 5 mA -1.0 mV/°C
VN Output Noise Voltage f = 10 Hz to 100 kHz, TA = +25°C 58.0 μV/VO
RR Ripple Rejection(9) f = 120 Hz, VI = 13 V to 23 V 56.0 71.0 dB
VDROP Dropout Voltage IO = 1 A, TJ = +25°C 2.0 V
RO Output Resistance(9) f = 1 kHz 17.0 mΩ
ISC Short-Circuit Current VI = 35 V, TJ = +25°C 250 mA
IPK Peak Current(9) TJ = +25°C 2.2 A
LM78XX / LM78XXA — 3-Terminal 1 A Positive Voltage Regulator
© 2006 Fairchild Semiconductor Corporation www.fairchildsemi.com
LM78XX / LM78XXA Rev. 1.3.0 7
Electrical Characteristics (LM7810)
Refer to the test circuit, -40°C < TJ < 125°C, IO = 500 mA, VI = 16 V, CI = 0.33 μF,CO = 0.1 μF, unless otherwise
specified.
Notes:
10. Load and line regulation are specified at constant junction temperature. Changes in VO due to heating effects must
be taken into account separately. Pulse testing with low duty is used.
11. These parameters, although guaranteed, are not 100% tested in production.
Symbol Parameter Conditions Min. Typ. Max. Unit
VO Output Voltage
TJ = +25°C 9.6 10.0 10.4
IO = 5 mA to 1 A, PO ≤ 15 W, V
VI = 12.5 V to 25 V 9.5 10.0 10.5
Regline Line Regulation(10) TJ = +25°C
VI = 12.5 V to 25 V 10 200
mV
VI = 13 V to 25 V 3 100
Regload Load Regulation(10) TJ = +25°C
IO = 5 mA to 1.5 A 12 200
mV
IO = 250 mA to 750 mA 4 400
IQ Quiescent Current TJ =+25°C 5.1 8.0 mA
ΔIQ
Quiescent Current
Change
IO = 5 mA to 1 A 0.5
mA
VI = 12.5 V to 29 V 1.0
ΔVO/ΔT Output Voltage Drift(11) IO = 5 mA -1.0 mV/°C
VN Output Noise Voltage f = 10 Hz to 100 kHz, TA = +25°C 58.0 μV/VO
RR Ripple Rejection(11) f = 120 Hz, VI = 13 V to 23 V 56.0 71.0 dB
VDROP Dropout Voltage IO = 1 A, TJ = +25°C 2.0 V
RO Output Resistance(11) f = 1 kHz 17.0 mΩ
ISC Short-Circuit Current VI = 35 V, TJ = +25°C 250 mA
IPK Peak Current(11) TJ = +25°C 2.2 A
LM78XX / LM78XXA — 3-Terminal 1 A Positive Voltage Regulator
© 2006 Fairchild Semiconductor Corporation www.fairchildsemi.com
LM78XX / LM78XXA Rev. 1.3.0 8
Electrical Characteristics (LM7812)
Refer to the test circuit, -40°C < TJ < 125°C, IO = 500 mA, VI = 19 V, CI = 0.33 μF,CO = 0.1 μF, unless otherwise
specified.
Notes:
12. Load and line regulation are specified at constant junction temperature. Changes in VO due to heating effects must
be taken into account separately. Pulse testing with low duty is used.
13. These parameters, although guaranteed, are not 100% tested in production.
Symbol Parameter Conditions Min. Typ. Max. Unit
VO Output Voltage
TJ = +25°C 11.5 12.0 12.5
IO = 5 mA to 1 A, PO ≤ 15 W, V
VI = 14.5 V to 27 V 11.4 12.0 12.6
Regline Line Regulation(12) TJ = +25°C
VI = 14.5 V to 30 V 10 240
mV
VI = 16 V to 22 V 3 120
Regload Load Regulation(12) TJ = +25°C
IO = 5 mA to 1.5 A 11 240
mV
IO = 250 mA to 750 mA 5 120
IQ Quiescent Current TJ =+25°C 5.1 8.0 mA
ΔIQ
Quiescent Current
Change
IO = 5 mA to 1 A 0.1 0.5
mA
VI = 14.5 V to 30 V 0.5 1.0
ΔVO/ΔT Output Voltage Drift(13) IO = 5 mA -1.0 mV/°C
VN Output Noise Voltage f = 10 Hz to 100 kHz, TA = +25°C 76.0 μV/VO
RR Ripple Rejection(13) f = 120 Hz, VI = 15 V to 25 V 55.0 71.0 dB
VDROP Dropout Voltage IO = 1 A, TJ = +25°C 2.0 V
RO Output Resistance(13) f = 1 kHz 18.0 mΩ
ISC Short-Circuit Current VI = 35 V, TJ = +25°C 230 mA
IPK Peak Current(13) TJ = +25°C 2.2 A
LM78XX / LM78XXA — 3-Terminal 1 A Positive Voltage Regulator
© 2006 Fairchild Semiconductor Corporation www.fairchildsemi.com
LM78XX / LM78XXA Rev. 1.3.0 9
Electrical Characteristics (LM7815)
Refer to the test circuit, -40°C < TJ < 125°C, IO = 500 mA, VI = 23 V, CI = 0.33 μF,CO = 0.1 μF, unless otherwise
specified.
Notes:
14. Load and line regulation are specified at constant junction temperature. Changes in VO due to heating effects must
be taken into account separately. Pulse testing with low duty is used.
15. These parameters, although guaranteed, are not 100% tested in production.
Symbol Parameter Conditions Min. Typ. Max. Unit
VO Output Voltage
TJ = +25°C 14.40 15.00 15.60
IO = 5 mA to 1 A, PO ≤ 15 W, V
VI = 17.5 V to 30 V 14.25 15.00 15.75
Regline Line Regulation(14) TJ = +25°C
VI = 17.5 V to 30 V 11 300
mV
VI = 20 V to 26 V 3 150
Regload Load Regulation(14) TJ = +25°C
IO = 5 mA to 1.5 A 12 300
mV
IO = 250 mA to 750 mA 4 150
IQ Quiescent Current TJ =+25°C 5.2 8.0 mA
ΔIQ
Quiescent Current
Change
IO = 5 mA to 1 A 0.5
mA
VI = 17.5 V to 30 V 1.0
ΔVO/ΔT Output Voltage Drift(15) IO = 5 mA -1.0 mV/°C
VN Output Noise Voltage f = 10 Hz to 100 kHz, TA = +25°C 90.0 μV/VO
RR Ripple Rejection(15) f = 120 Hz, VI = 18.5 V to 28.5 V 54.0 70.0 dB
VDROP Dropout Voltage IO = 1 A, TJ =+25°C 2.0 V
RO Output Resistance(15) f = 1 kHz 19.0 mΩ
ISC Short-Circuit Current VI = 35 V, TJ = +25°C 250 mA
IPK Peak Current(15) TJ = +25°C 2.2 A
LM78XX / LM78XXA — 3-Terminal 1 A Positive Voltage Regulator
© 2006 Fairchild Semiconductor Corporation www.fairchildsemi.com
LM78XX / LM78XXA Rev. 1.3.0 10
Electrical Characteristics (LM7818)
Refer to the test circuit, -40°C < TJ < 125°C, IO = 500 mA, VI = 27 V, CI = 0.33 μF, CO = 0.1 μF, unless otherwise
specified.
Notes:
16. Load and line regulation are specified at constant junction temperature. Changes in VO due to heating effects must
be taken into account separately. Pulse testing with low duty is used.
17. These parameters, although guaranteed, are not 100% tested in production.
Symbol Parameter Conditions Min. Typ. Max. Unit
VO Output Voltage
TJ = +25°C 17.3 18.0 18.7
IO = 5 mA to 1 A, PO ≤ 15 W, V
VI = 21 V to 33 V 17.1 18.0 18.9
Regline Line Regulation(16) TJ = +25°C
VI = 21 V to 33 V 15 360
mV
VI = 24 V to 30 V 5 180
Regload Load Regulation(16) TJ = +25°C
IO = 5 mA to 1.5 A 15 360
mV
IO = 250 mA to 750 mA 5 180
IQ Quiescent Current TJ =+25°C 5.2 8.0 mA
ΔIQ
Quiescent Current
Change
IO = 5 mA to 1 A 0.5
mA
VI = 21 V to 33 V 1.0
ΔVO/ΔT Output Voltage Drift(17) IO = 5 mA -1.0 mV/°C
VN Output Noise Voltage f = 10 Hz to 100 kHz, TA = +25°C 110 μV/VO
RR Ripple Rejection(17) f = 120 Hz, VI = 22 V to 32 V 53.0 69.0 dB
VDROP Dropout Voltage IO = 1 A, TJ =+25°C 2.0 V
RO Output Resistance(17) f = 1 kHz 22.0 mΩ
ISC Short-Circuit Current VI = 35 V, TJ =+25°C 250 mA
IPK Peak Current(17) TJ =+25°C 2.2 A
LM78XX / LM78XXA — 3-Terminal 1 A Positive Voltage Regulator
© 2006 Fairchild Semiconductor Corporation www.fairchildsemi.com
LM78XX / LM78XXA Rev. 1.3.0 11
Electrical Characteristics (LM7824)
Refer to the test circuit, -40°C < TJ < 125°C, IO = 500 mA, VI = 33 V, CI = 0.33 μF, CO = 0.1 μF, unless otherwise
specified.
Notes:
18. Load and line regulation are specified at constant junction temperature. Changes in VO due to heating effects must
be taken into account separately. Pulse testing with low duty is used.
19. These parameters, although guaranteed, are not 100% tested in production.
Symbol Parameter Conditions Min. Typ. Max. Unit
VO Output Voltage
TJ = +25°C 23.00 24.00 25.00
IO = 5 mA to 1 A, PO ≤ 15 W, V
VI = 27 V to 38 V 22.80 24.00 25.25
Regline Line Regulation(18) TJ = +25°C
VI = 27 V to 38 V 17 480
mV
VI = 30 V to 36 V 6 240
Regload Load Regulation(18) TJ = +25°C
IO = 5 mA to 1.5 A 15 480
mV
IO = 250 mA to 750 mA 5 240
IQ Quiescent Current TJ =+25°C 5.2 8.0 mA
ΔIQ
Quiescent Current
Change
IO = 5 mA to 1 A 0.1 0.5
mA
VI = 27 V to 38 V 0.5 1.0
ΔVO/ΔT Output Voltage Drift(19) IO = 5 mA -1.5 mV/°C
VN Output Noise Voltage f = 10 Hz to 100 kHz, TA = +25°C 6.0 μV/VO
RR Ripple Rejection(19) f = 120 Hz, VI = 28 V to 38 V 50.0 67.0 dB
VDROP Dropout Voltage IO = 1 A, TJ = +25°C 2.0 V
RO Output Resistance(19) f = 1 kHz 28.0 mΩ
ISC Short-Circuit Current VI = 35 V, TJ = +25°C 230 mA
IPK Peak Current(19) TJ = +25°C 2.2 A
LM78XX / LM78XXA — 3-Terminal 1 A Positive Voltage Regulator
© 2006 Fairchild Semiconductor Corporation www.fairchildsemi.com
LM78XX / LM78XXA Rev. 1.3.0 12
Electrical Characteristics (LM7805A)
Refer to the test circuit, 0°C < TJ < 125°C, IO = 1 A, VI = 10 V, CI = 0.33 μF, CO = 0.1 μF, unless otherwise specified.
Notes:
20. Load and line regulation are specified at constant junction temperature. Changes in VO due to heating effects must
be taken into account separately. Pulse testing with low duty is used.
21. These parameters, although guaranteed, are not 100% tested in production.
Symbol Parameter Conditions Min. Typ. Max. Unit
VO Output Voltage
TJ = +25°C 4.9 5.0 5.1
IO = 5 mA to 1 A, PO ≤ 15 W, V
VI = 7.5 V to 20 V 4.8 5.0 5.2
Regline Line Regulation(20)
VI = 7.5 V to 25 V, IO = 500 mA 5.0 50.0
mV
VI = 8 V to 12 V 3.0 50.0
TJ = +25°C
VI = 7.3 V to 20 V 5.0 50.0
VI = 8 V to 12 V 1.5 25.0
Regload Load Regulation(20)
TJ = +25°C, IO = 5 mA to 1.5 A 9.0 100.0
IO = 5 mA to 1 A 9.0 100.0 mV
IO = 250 mA to 750 mA 4.0 50.0
IQ Quiescent Current TJ =+25°C 5.0 6.0 mA
ΔIQ
Quiescent Current
Change
IO = 5 mA to 1 A 0.5
VI = 8 V to 25 V, IO = 500 mA 0.8 mA
VI = 7.5 V to 20 V, TJ = +25°C 0.8
ΔVO/ΔT Output Voltage Drift(21) IO = 5 mA -0.8 mV/°C
VN Output Noise Voltage f = 10 Hz to 100 kHz, TA = +25°C 10.0 μV/VO
RR Ripple Rejection(21) f = 120 Hz, VO = 500 mA,
VI =8 V to 18 V 68.0 dB
VDROP Dropout Voltage IO = 1 A, TJ =+25°C 2.0 V
RO Output Resistance(21) f = 1 kHz 17.0 mΩ
ISC Short-Circuit Current VI = 35 V, TJ =+25°C 250 mA
IPK Peak Current(21) TJ =+25°C 2.2 A
LM78XX / LM78XXA — 3-Terminal 1 A Positive Voltage Regulator
© 2006 Fairchild Semiconductor Corporation www.fairchildsemi.com
LM78XX / LM78XXA Rev. 1.3.0 13
Electrical Characteristics (LM7809A)
Refer to the test circuit, 0°C < TJ < 125°C, IO = 1 A, VI = 15 V, CI = 0.33 μF,CO = 0.1 μF, unless otherwise specified.
Notes:
22. Load and line regulation are specified at constant junction temperature. Changes in VO due to heating effects must
be taken into account separately. Pulse testing with low duty is used.
23. These parameters, although guaranteed, are not 100% tested in production.
Symbol Parameter Conditions Min. Typ. Max. Unit
VO Output Voltage
TJ = +25°C 8.82 9.00 9.16
IO = 5 mA to 1 A, PO ≤ 15 W, V
VI = 11.2 V to 24 V 8.65 9.00 9.35
Regline Line Regulation(22)
VI = 11.7 V to 25 V, IO = 500 mA 6.0 90.0
mV
VI = 12.5 V to 19 V 4.0 45.0
TJ = +25°C
VI = 11.5 V to 24 V 6.0 90.0
VI = 12.5 V to 19 V 2.0 45.0
Regload Load Regulation(22)
TJ = +25°C, IO = 5 mA to 1.5 A 12.0 100.0
IO = 5 mA to 1 A 12.0 100.0 mV
IO = 250 mA to 750 mA 5.0 50.0
IQ Quiescent Current TJ = +25°C 5.0 6.0 mA
ΔIQ
Quiescent Current
Change
IO = 5 mA to 1 A 0.5
VI = 12 V to 25 V, IO = 500 mA 0.8 mA
VI = 11.7 V to 25 V, TJ = +25°C 0.8
ΔVO/ΔT Output Voltage Drift(23) IO = 5 mA -1.0 mV/°C
VN Output Noise Voltage f = 10 Hz to 100 kHz, TA = +25°C 10.0 μV/VO
RR Ripple Rejection(23) f = 120 Hz, VO = 500 mA,
VI =12 V to 22 V 62.0 dB
VDROP Dropout Voltage IO = 1 A, TJ = +25°C 2.0 V
RO Output Resistance(23) f = 1 kHz 17.0 mΩ
ISC Short-Circuit Current VI = 35 V, TJ = +25°C 250 mA
IPK Peak Current(23) TJ = +25°C 2.2 A
LM78XX / LM78XXA — 3-Terminal 1 A Positive Voltage Regulator
© 2006 Fairchild Semiconductor Corporation www.fairchildsemi.com
LM78XX / LM78XXA Rev. 1.3.0 14
Electrical Characteristics (LM7810A)
Refer to the test circuit, 0°C < TJ < 125°C, IO = 1 A, VI = 16 V, CI = 0.33 μF, CO = 0.1 μF, unless otherwise specified.
Notes:
24. Load and line regulation are specified at constant junction temperature. Changes in VO due to heating effects must
be taken into account separately. Pulse testing with low duty is used.
25. These parameters, although guaranteed, are not 100% tested in production.
Symbol Parameter Conditions Min. Typ. Max. Unit
VO Output Voltage
TJ = +25°C 9.8 10.0 10.2
IO = 5 mA to 1 A, PO ≤ 15 W, V
VI = 12.8 V to 25 V 9.6 10.0 10.4
Regline Line Regulation(24)
VI = 12.8 V to 26 V, IO = 500 mA 8.0 100.0
mV
VI = 13 V to 20 V 4.0 50.0
TJ = +25°C
VI = 12.5 V to 25 V 8.0 100.0
VI = 13 V to 20 V 3.0 50.0
Regload Load Regulation(24)
TJ = +25°C, IO = 5 mA to 1.5 A 12.0 100.0
IO = 5 mA to 1 A 12.0 100.0 mV
IO = 250 mA to 750 mA 5.0 50.0
IQ Quiescent Current TJ =+25°C 5.0 6.0 mA
ΔIQ
Quiescent Current
Change
IO = 5 mA to 1 A 0.5
VI = 12.8 V to 25 V, IO = 500 mA 0.8 mA
VI = 13 V to 26 V, TJ = +25°C 0.5
ΔVO/ΔT Output Voltage Drift(25) IO = 5 mA -1.0 mV/°C
VN Output Noise Voltage f = 10 Hz to 100 kHz, TA = +25°C 10.0 μV/VO
RR Ripple Rejection(25) f = 120 Hz, VO = 500 mA,
VI =14 V to 24 V 62.0 dB
VDROP Dropout Voltage IO = 1 A, TJ =+25°C 2.0 V
RO Output Resistance(25) f = 1 kHz 17.0 mΩ
ISC Short-Circuit Current VI = 35 V, TJ =+25°C 250 mA
IPK Peak Current(25) TJ =+25°C 2.2 A
LM78XX / LM78XXA — 3-Terminal 1 A Positive Voltage Regulator
© 2006 Fairchild Semiconductor Corporation www.fairchildsemi.com
LM78XX / LM78XXA Rev. 1.3.0 15
Electrical Characteristics (LM7812A)
Refer to the test circuit, 0°C < TJ < 125°C, IO = 1 A, VI = 19 V, CI = 0.33 μF, CO = 0.1 μF, unless otherwise specified.
Notes:
26. Load and line regulation are specified at constant junction temperature. Changes in VO due to heating effects must
be taken into account separately. Pulse testing with low duty is used.
27. These parameters, although guaranteed, are not 100% tested in production.
Symbol Parameter Conditions Min. Typ. Max. Unit
VO Output Voltage
TJ = +25°C 11.75 12.00 12.25
IO = 5 mA to 1 A, PO ≤ 15 W, V
VI = 14.8 V to 27 V 11.50 12.00 12.50
Regline Line Regulation(26)
VI = 14.8 V to 30 V, IO = 500 mA 10.0 120.0
mV
VI = 16 V to 22 V 4.0 120.0
TJ = +25°C
VI = 14.5 V to 27 V 10.0 120.0
VI = 16 V to 22 V 3.0 60.0
Regload Load Regulation(26)
TJ = +25°C, IO = 5 mA to 1.5 A 12.0 100.0
IO = 5 mA to 1 A 12.0 100.0 mV
IO = 250 mA to 750 mA 5.0 50.0
IQ Quiescent Current TJ = +25°C 5.0 6.0 mA
ΔIQ
Quiescent Current
Change
IO = 5 mA to 1 A 0.5
VI = 14 V to 27 V, IO = 500 mA 0.8 mA
VI = 15 V to 30 V, TJ = +25°C 0.8
ΔVO/ΔT Output Voltage Drift(27) IO = 5 mA -1.0 mV/°C
VN Output Noise Voltage f = 10 Hz to 100 kHz, TA = +25°C 10.0 μV/VO
RR Ripple Rejection(27) f = 120 Hz, VO = 500 mA,
VI =14 V to 24 V 60.0 dB
VDROP Dropout Voltage IO = 1 A, TJ = +25°C 2.0 V
RO Output Resistance(27) f = 1 kHz 18.0 mΩ
ISC Short-Circuit Current VI = 35 V, TJ = +25°C 250 mA
IPK Peak Current(27) TJ = +25°C 2.2 A
LM78XX / LM78XXA — 3-Terminal 1 A Positive Voltage Regulator
© 2006 Fairchild Semiconductor Corporation www.fairchildsemi.com
LM78XX / LM78XXA Rev. 1.3.0 16
Electrical Characteristics (LM7815A)
Refer to the test circuit, 0°C < TJ < 125°C, IO = 1 A, VI = 23 V, CI = 0.33 μF, CO = 0.1 μF, unless otherwise specified.
Notes:
28. Load and line regulation are specified at constant junction temperature. Changes in VO due to heating effects must
be taken into account separately. Pulse testing with low duty is used.
29. These parameters, although guaranteed, are not 100% tested in production.
Symbol Parameter Conditions Min. Typ. Max. Unit
VO Output Voltage
TJ = +25°C 14.75 15.00 15.30
IO = 5 mA to 1 A, PO ≤ 15 W, V
VI = 17.7 V to 30 V 14.40 15.00 15.60
Regline Line Regulation(28)
VI = 17.4 V to 30 V, IO = 500 mA 10.0 150.0
mV
VI = 20 V to 26 V 5.0 150.0
TJ = +25°C
VI = 17.5 V to 30 V 11.0 150.0
VI = 20 V to 26 V 3.0 75.0
Regload Load Regulation(28)
TJ = +25°C, IO = 5 mA to 1.5 A 12.0 100.0
IO = 5 mA to 1 A 12.0 100.0 mV
IO = 250 mA to 750 mA 5.0 50.0
IQ Quiescent Current TJ =+25°C 5.2 6.0 mA
ΔIQ
Quiescent Current
Change
IO = 5 mA to 1 A 0.5
VI = 17.5 V to 30 V, IO = 500 mA 0.8 mA
VI = 17.5 V to 30 V, TJ = +25°C 0.8
ΔVO/ΔT Output Voltage Drift(29) IO = 5 mA -1.0 mV/°C
VN Output Noise Voltage f = 10 Hz to 100 kHz, TA = +25°C 10.0 μV/VO
RR Ripple Rejection(29) f = 120 Hz, VO = 500 mA,
VI =18.5 V to 28.5 V 58.0 dB
VDROP Dropout Voltage IO = 1 A, TJ =+25°C 2.0 V
RO Output Resistance(29) f = 1 kHz 19.0 mΩ
ISC Short-Circuit Current VI = 35 V, TJ =+25°C 250 mA
IPK Peak Current(29) TJ =+25°C 2.2 A
LM78XX / LM78XXA — 3-Terminal 1 A Positive Voltage Regulator
© 2006 Fairchild Semiconductor Corporation www.fairchildsemi.com
LM78XX / LM78XXA Rev. 1.3.0 17
Typical Performance Characteristics
Figure 2. Quiescent Current Figure 3. Peak Output Current
Figure 4. Output Voltage Figure 5. Quiescent Current
LM78XX / LM78XXA — 3-Terminal 1 A Positive Voltage Regulator
© 2006 Fairchild Semiconductor Corporation www.fairchildsemi.com
LM78XX / LM78XXA Rev. 1.3.0 18
Typical Applications
Figure 6. DC Parameters
Figure 7. Load Regulation
Figure 8. Ripple Rejection
CI CO 0.1μF
0.33μF
Input Output
LM78XX
1 3
2
LM78XX
3
2
1
0.33μF
270pF
100Ω 30μS
RL
2N6121
or EQ
Input Output
VO
0V
VO
LM78XX
Input Output
5.1Ω
0.33μF
2
1 3
RL
470μF
120Hz +
LM78XX / LM78XXA — 3-Terminal 1 A Positive Voltage Regulator
© 2006 Fairchild Semiconductor Corporation www.fairchildsemi.com
LM78XX / LM78XXA Rev. 1.3.0 19
Figure 9. Fixed-Output Regulator
Notes:
29. To specify an output voltage, substitute voltage value for “XX”. A common ground is required between the input and
the output voltage. The input voltage must remain typically 2.0 V above the output voltage even during the low point on
the input ripple voltage.
30. CI is required if regulator is located an appreciable distance from power supply filter.
31. CO improves stability and transient response.
Figure 10.
Figure 11. Circuit for Increasing Output Voltage
CI CO 0.1μF
0.33μF
Input Output
LM78XX
1 3
2
CI CO 0.1μF
0.33μF
Output
Input
LM78XX
1 3
2 VXX
R1
RL
IQ
IO
IO = R1 +IQ
VXX
CI CO 0.1μF
0.33μF
Output
Input
LM78XX
1 3
2 VXX
R1
R2
IQ
IRI ≥ 5 IQ
VO = VXX(1 + R2 / R1) + IQR2
LM78XX / LM78XXA — 3-Terminal 1 A Positive Voltage Regulator
© 2006 Fairchild Semiconductor Corporation www.fairchildsemi.com
LM78XX / LM78XXA Rev. 1.3.0 20
Figure 12. Adjustable Output Regulator (7 V to 30 V)
Figure 13. High-Current Voltage Regulator
Figure 14. High Output Current with Short-Circuit Protection
LM741
-
+
2
3
6
4
2
1 3
CI 0.33μF
Input Output
0.1μF
CO
LM7805
10kΩ
IRI ≥ 5 IQ
VO = VXX(1 + R2 / R1) + IQR2
3
2
1
LM78XX
Output
Input
R1
3Ω
0.33μF
IREG
0.1μF
IO
IQ1
IO = IREG + BQ1 (IREG–VBEQ1/R1)
Q1 BD536
R1 =
VBEQ1
IREG–IQ1/ BQ1
LM78XX
Output
0.33μF 0.1μF
R1
3Ω
3
2
1
Input Q1
Q2
Q1 = TIP42
Q2 = TIP42
RSC =
I SC
VBEQ2
RSC
LM78XX / LM78XXA — 3-Terminal 1 A Positive Voltage Regulator
© 2006 Fairchild Semiconductor Corporation www.fairchildsemi.com
LM78XX / LM78XXA Rev. 1.3.0 21
Figure 15. Tracking Voltage Regulator
Figure 16. Split Power Supply (±15 V - 1 A)
LM78XX
LM741
0.33μF 0.1μF
1
2
3
7 2
6
4 3 4.7kΩ
4.7kΩ
TIP42
COMMON
COMMON
VO
-VO
VI
-VIN
_
+
1 3
2
1
2 3
0.33μF 0.1μF
2.2μF
1μF +
+
1N4001
1N4001
+15V
-15V
+20V
-20V
LM7815
MC7915
LM78XX / LM78XXA — 3-Terminal 1 A Positive Voltage Regulator
© 2006 Fairchild Semiconductor Corporation www.fairchildsemi.com
LM78XX / LM78XXA Rev. 1.3.0 22
Figure 17. Negative Output Voltage Circuit
Figure 18. Switching Regulator
LM78XX
Output
Input
+
1
2
0.1μF
3
LM78XX
1mH
1 3
2
2000μF
Input Output D45H11
0.33μF
470Ω
4.7Ω
10μF
0.5Ω
Z1
+
+
LM78XX / LM78XXA — 3-Terminal 1 A Positive Voltage Regulator
© 2006 Fairchild Semiconductor Corporation www.fairchildsemi.com
LM78XX / LM78XXA Rev. 1.3.0 23
Physical Dimensions
Figure 19. TO-220, MOLDED, 3-LEAD, JEDEC VARIATION AB (ACTIVE)
Package drawings are provided as a service to customers considering Fairchild components. Drawings may change in any manner
without notice. Please note the revision and/or date on the drawing and contact a Fairchild Semiconductor representative to verify or
obtain the most recent revision. Package specifications do not expand the terms of Fairchild’s worldwide terms and conditions, specifically the
warranty therein, which covers Fairchild products.
Always visit Fairchild Semiconductor’s online packaging area for the most recent package drawings:
http://www.fairchildsemi.com/dwg/TO/TO220B03.pdf.
For current tape and reel specifications, visit Fairchild Semiconductor’s online packaging area:
http://www.fairchildsemi.com/packing_dwg/PKG-TO220B03_TC.pdf.
TO-220 (SINGLE GAUGE)
© Fairchild Semiconductor Corporation www.fairchildsemi.com
TRADEMARKS
The following includes registered and unregistered trademarks and service marks, owned by Fairchild Semiconductor and/or its global subsidiaries, and is not
intended to be an exhaustive list of all such trademarks.
2Cool
AccuPower
AX-CAP®*
BitSiC
Build it Now
CorePLUS
CorePOWER
CROSSVOLT
CTL
Current Transfer Logic
DEUXPEED®
Dual Cool™
EcoSPARK®
EfficientMax
ESBC
Fairchild®
Fairchild Semiconductor®
FACT Quiet Series
FACT®
FAST®
FastvCore
FETBench
FPS
F-PFS
FRFET®
Global Power ResourceSM
GreenBridge
Green FPS
Green FPS e-Series
Gmax
GTO
IntelliMAX
ISOPLANAR
Making Small Speakers Sound Louder
and Better™
MegaBuck
MICROCOUPLER
MicroFET
MicroPak
MicroPak2
MillerDrive
MotionMax
mWSaver®
OptoHiT
OPTOLOGIC®
OPTOPLANAR®
®
PowerTrench®
PowerXS™
Programmable Active Droop
QFET®
QS
Quiet Series
RapidConfigure
Saving our world, 1mW/W/kW at a time™
SignalWise
SmartMax
SMART START
Solutions for Your Success
SPM®
STEALTH
SuperFET®
SuperSOT-3
SuperSOT-6
SuperSOT-8
SupreMOS®
SyncFET
Sync-Lock™
®*
TinyBoost®
TinyBuck®
TinyCalc
TinyLogic®
TINYOPTO
TinyPower
TinyPWM
TinyWire
TranSiC
TriFault Detect
TRUECURRENT®*
SerDes
UHC®
Ultra FRFET
UniFET
VCX
VisualMax
VoltagePlus
XS™
* Trademarks of System General Corporation, used under license by Fairchild Semiconductor.
DISCLAIMER
FAIRCHILD SEMICONDUCTOR RESERVES THE RIGHT TO MAKE CHANGES WITHOUT FURTHER NOTICE TO ANY PRODUCTS HEREIN TO IMPROVE
RELIABILITY, FUNCTION, OR DESIGN. FAIRCHILD DOES NOT ASSUME ANY LIABILITY ARISING OUT OF THE APPLICATION OR USE OF ANY PRODUCT
OR CIRCUIT DESCRIBED HEREIN; NEITHER DOES IT CONVEY ANY LICENSE UNDER ITS PATENT RIGHTS, NOR THE RIGHTS OF OTHERS. THESE
SPECIFICATIONS DO NOT EXPAND THE TERMS OF FAIRCHILD’S WORLDWIDE TERMS AND CONDITIONS, SPECIFICALLY THE WARRANTY THEREIN,
WHICH COVERS THESE PRODUCTS.
LIFE SUPPORT POLICY
FAIRCHILD’S PRODUCTS ARE NOT AUTHORIZED FOR USE AS CRITICAL COMPONENTS IN LIFE SUPPORT DEVICES OR SYSTEMS WITHOUT THE
EXPRESS WRITTEN APPROVAL OF FAIRCHILD SEMICONDUCTOR CORPORATION.
As used herein:
1. Life support devices or systems are devices or systems which, (a) are
intended for surgical implant into the body or (b) support or sustain
life, and (c) whose failure to perform when properly used in
accordance with instructions for use provided in the labeling, can be
reasonably expected to result in a significant injury of the user.
2. A critical component in any component of a life support, device, or
system whose failure to perform can be reasonably expected to
cause the failure of the life support device or system, or to affect its
safety or effectiveness.
ANTI-COUNTERFEITING POLICY
Fairchild Semiconductor Corporation's Anti-Counterfeiting Policy. Fairchild's Anti-Counterfeiting Policy is also stated on our external website, www.fairchildsemi.com,
under Sales Support.
Counterfeiting of semiconductor parts is a growing problem in the industry. All manufacturers of semiconductor products are experiencing counterfeiting of their
parts. Customers who inadvertently purchase counterfeit parts experience many problems such as loss of brand reputation, substandard performance, failed
applications, and increased cost of production and manufacturing delays. Fairchild is taking strong measures to protect ourselves and our customers from the
proliferation of counterfeit parts. Fairchild strongly encourages customers to purchase Fairchild parts either directly from Fairchild or from Authorized Fairchild
Distributors who are listed by country on our web page cited above. Products customers buy either from Fairchild directly or from Authorized Fairchild Distributors
are genuine parts, have full traceability, meet Fairchild's quality standards for handling and storage and provide access to Fairchild's full range of up-to-date technical
and product information. Fairchild and our Authorized Distributors will stand behind all warranties and will appropriately address any warranty issues that may arise.
Fairchild will not provide any warranty coverage or other assistance for parts bought from Unauthorized Sources. Fairchild is committed to combat this global
problem and encourage our customers to do their part in stopping this practice by buying direct or from authorized distributors.
PRODUCT STATUS DEFINITIONS
Definition of Terms
Datasheet Identification Product Status Definition
Advance Information Formative / In Design Datasheet contains the design specifications for product development. Specifications may change
in any manner without notice.
Preliminary First Production Datasheet contains preliminary data; supplementary data will be published at a later date. Fairchild
Semiconductor reserves the right to make changes at any time without notice to improve design.
No Identification Needed Full Production Datasheet contains final specifications. Fairchild Semiconductor reserves the right to make
changes at any time without notice to improve the design.
Obsolete Not In Production Datasheet contains specifications on a product that is discontinued by Fairchild Semiconductor.
The datasheet is for reference information only.
Rev. I65
®
Low Cost Low Power
Instrumentation Amplifier
AD620
Rev. H
Information furnished by Analog Devices is believed to be accurate and reliable.
However, no responsibility is assumed by Analog Devices for its use, nor for any
infringements of patents or other rights of third parties that may result from its use.
Specifications subject to change without notice. No license is granted by implication
or otherwise under any patent or patent rights of Analog Devices. Trademarks and
registered trademarks are the property of their respective owners.
One Technology Way, P.O. Box 9106, Norwood, MA 02062-9106, U.S.A.
Tel: 781.329.4700 www.analog.com
Fax: 781.326.8703© 2003–2011 Analog Devices, Inc. All rights reserved.
FEATURES
Easy to use
Gain set with one external resistor
(Gain range 1 to 10,000)
Wide power supply range (±2.3 V to ±18 V)
Higher performance than 3 op amp IA designs
Available in 8-lead DIP and SOIC packaging
Low power, 1.3 mA max supply current
Excellent dc performance (B grade)
50 μV max, input offset voltage
0.6 μV/°C max, input offset drift
1.0 nA max, input bias current
100 dB min common-mode rejection ratio (G = 10)
Low noise
9 nV/√Hz @ 1 kHz, input voltage noise
0.28 μV p-p noise (0.1 Hz to 10 Hz)
Excellent ac specifications
120 kHz bandwidth (G = 100)
15 μs settling time to 0.01%
APPLICATIONS
Weigh scales
ECG and medical instrumentation
Transducer interface
Data acquisition systems
Industrial process controls
Battery-powered and portable equipment
CONNECTION DIAGRAM
–IN
RG
–VS
+IN
RG
+VS
OUTPUT
REF
1
2
3
4
8
7
6
AD620 5
TOP VIEW
00775-0-001
Figure 1. 8-Lead PDIP (N), CERDIP (Q), and SOIC (R) Packages
PRODUCT DESCRIPTION
The AD620 is a low cost, high accuracy instrumentation
amplifier that requires only one external resistor to set gains of
1 to 10,000. Furthermore, the AD620 features 8-lead SOIC and
DIP packaging that is smaller than discrete designs and offers
lower power (only 1.3 mA max supply current), making it a
good fit for battery-powered, portable (or remote) applications.
The AD620, with its high accuracy of 40 ppm maximum
nonlinearity, low offset voltage of 50 μV max, and offset drift of
0.6 μV/°C max, is ideal for use in precision data acquisition
systems, such as weigh scales and transducer interfaces.
Furthermore, the low noise, low input bias current, and low power
of the AD620 make it well suited for medical applications, such
as ECG and noninvasive blood pressure monitors.
The low input bias current of 1.0 nA max is made possible with
the use of Superϐeta processing in the input stage. The AD620
works well as a preamplifier due to its low input voltage noise of
9 nV/√Hz at 1 kHz, 0.28 μV p-p in the 0.1 Hz to 10 Hz band,
and 0.1 pA/√Hz input current noise. Also, the AD620 is well
suited for multiplexed applications with its settling time of 15 μs
to 0.01%, and its cost is low enough to enable designs with one
in-amp per channel.
Table 1. Next Generation Upgrades for AD620
Part Comment
AD8221 Better specs at lower price
AD8222 Dual channel or differential out
AD8226 Low power, wide input range
AD8220 JFET input
AD8228 Best gain accuracy
AD8295 +2 precision op amps or differential out
AD8429 Ultra low noise
0 5 10 15 20
30,000
5,000
10,000
15,000
20,000
25,000
0
TOTAL ERROR, PPM OF FULL SCALE
SUPPLY CURRENT (mA)
AD620A
RG
3 OP AMP
IN-AMP
(3 OP-07s)
00775-0-002
Figure 2. Three Op Amp IA Designs vs. AD620
IMPORTANT LINKS for the AD620*
Last content update 01/08/2014 09:49 am
Looking for a high performance in-amp with lower noise, wider bandwidth, and fast settling time? Consider the AD8421
Looking for a high performance in-amp with lower power and a rail-to-rail output? Consider the AD8422.
DOCUMENTATION
AD620: Military Data Sheet
AN-282: Fundamentals of Sampled Data Systems
AN-244: A User's Guide to I.C. Instrumentation Amplifiers
AN-245: Instrumentation Amplifiers Solve Unusual Design Problems
AN-671: Reducing RFI Rectification Errors in In-Amp Circuits
AN-589: Ways to Optimize the Performance of a Difference Amplifier
A Designer's Guide to Instrumentation Amplifiers (3rd Edition)
UG-261: Evaluation Boards for the AD62x, AD822x and AD842x Series
ECG Front-End Design is Simplified with MicroConverter
Low-Power, Low-Voltage IC Choices for ECG System Requirements
Ask The Applications Engineer-10
Auto-Zero Amplifiers
High-performance Adder Uses Instrumentation Amplifiers
Protecting Instrumentation Amplifiers
Input Filter Prevents Instrumentation-amp RF-Rectification Errors
The AD8221 - Setting a New Industry Standard for Instrumentation
Amplifiers
ADI Warns Against Misuse of COTS Integrated Circuits
Space Qualified Parts List
Applying Instrumentation Amplifiers Effectively: The Importance of an
Input Ground Return
Leading Inside Advertorials: Applying Instrumentation Amplifiers
Effectively–The Importance of an Input Ground Return
DESIGN TOOLS, MODELS, DRIVERS & SOFTWARE
In-Amp Error Calculator
These tools will help estimate error contributions in your
instrumentation amplifier circuit. It uses input parameters such as
temperature, gain, voltage input, and source impedance to determine
the errors that can contribute to your overall design.
In-Amp Common Mode Calculator
AD620 SPICE Macro-Model
AD620A SPICE Macro-Model
AD620B SPICE Macro-Model
AD620S SPICE Macro-Model
AD620 SABER Macro-Model Conv, 10/00
EVALUATION KITS & SYMBOLS & FOOTPRINTS
View the Evaluation Boards and Kits page for documentation and
purchasing
Symbols and Footprints
PRODUCT RECOMMENDATIONS & REFERENCE DESIGNS
CN-0146: Low Cost Programmable Gain Instrumentation Amplifier
Circuit Using the ADG1611 Quad SPST Switch and AD620
Instrumentation Amplifier
DESIGN COLLABORATION COMMUNITY
Collaborate Online with the ADI support team and other designers
about select ADI products.
Follow us on Twitter: www.twitter.com/ADI_News
Like us on Facebook: www.facebook.com/AnalogDevicesInc
DESIGN SUPPORT
Submit your support request here:
Linear and Data Converters
Embedded Processing and DSP
Telephone our Customer Interaction Centers toll free:
Americas: 1-800-262-5643
Europe: 00800-266-822-82
China: 4006-100-006
India: 1800-419-0108
Russia: 8-800-555-45-90
Quality and Reliability
Lead(Pb)-Free Data
SAMPLE & BUY
AD620
View Price & Packaging
Request Evaluation Board
Request Samples Check Inventory & Purchase
Find Local Distributors
* This page was dynamically generated by Analo g Devices, Inc. and inserted into this data sheet.
Note: Dynamic changes to the content on this page (labeled 'Important Links') does not
constitute a change to the revision number of the product data sheet.
This content may be frequently modified.
Powered by TCPDF (www.tcpdf.org)
AD620
Rev. H | Page 2 of 20
TABLE OF CONTENTS
Specifications .....................................................................................3
Absolute Maximum Ratings ............................................................5
ESD Caution ..................................................................................5
Typical Performance Characteristics..............................................6
Theory of Operation.......................................................................12
Gain Selection..............................................................................15
Input and Output Offset Voltage ..............................................15
Reference Terminal .....................................................................15
Input Protection ..........................................................................15
RF Interference............................................................................15
Common-Mode Rejection.........................................................16
Grounding....................................................................................16
Ground Returns for Input Bias Currents.................................17
AD620ACHIPS Information.........................................................18
Outline Dimensions........................................................................19
Ordering Guide ...........................................................................20
REVISION HISTORY
7/11—Rev. G to Rev. H
Deleted Figure 3.................................................................................1
Added Table 1 ....................................................................................1
Moved Figure 2 ..................................................................................1
Added ESD Input Diodes to Simplified Schematic ....................12
Changes to Input Protection Section............................................15
Added Figure 41; Renumbered Sequentially ...............................15
Changes to AD620ACHIPS Information Section ......................18
Updated Ordering Guide ...............................................................20
12/04—Rev. F to Rev. G
Updated Format..................................................................Universal
Change to Features............................................................................1
Change to Product Description.......................................................1
Changes to Specifications.................................................................3
Added Metallization Photograph....................................................4
Replaced Figure 4-Figure 6 ..............................................................6
Replaced Figure 15............................................................................7
Replaced Figure 33..........................................................................10
Replaced Figure 34 and Figure 35.................................................10
Replaced Figure 37..........................................................................10
Changes to Table 3 ..........................................................................13
Changes to Figure 41 and Figure 42 .............................................14
Changes to Figure 43 ......................................................................15
Change to Figure 44 ........................................................................17
Changes to Input Protection section ............................................15
Deleted Figure 9 ..............................................................................15
Changes to RF Interference section..............................................15
Edit to Ground Returns for Input Bias Currents section...........17
Added AD620CHIPS to Ordering Guide ....................................19
7/03—Data Sheet Changed from Rev. E to Rev. F
Edit to FEATURES............................................................................1
Changes to SPECIFICATIONS.......................................................2
Removed AD620CHIPS from ORDERING GUIDE ...................4
Removed METALLIZATION PHOTOGRAPH...........................4
Replaced TPCs 1–3 ...........................................................................5
Replaced TPC 12...............................................................................6
Replaced TPC 30...............................................................................9
Replaced TPCs 31 and 32...............................................................10
Replaced Figure 4............................................................................10
Changes to Table I...........................................................................11
Changes to Figures 6 and 7 ............................................................12
Changes to Figure 8 ........................................................................13
Edited INPUT PROTECTION section........................................13
Added new Figure 9........................................................................13
Changes to RF INTERFACE section ............................................14
Edit to GROUND RETURNS FOR INPUT BIAS CURRENTS
section...............................................................................................15
Updated OUTLINE DIMENSIONS.............................................16
AD620
Rev. H | Page 3 of 20
SPECIFICATIONS
Typical @ 25°C, VS = ±15 V, and RL = 2 kΩ, unless otherwise noted.
Table 2.
Parameter Conditions
AD620A AD620B AD620S1
Min Typ Max Min Typ Max Min Typ Max Unit
GAIN G = 1 + (49.4 kΩ/RG)
Gain Range 1 10,000 1 10,000 1 10,000
Gain Error2 VOUT = ±10 V
G = 1 0.03 0.10 0.01 0.02 0.03 0.10 %
G = 10 0.15 0.30 0.10 0.15 0.15 0.30 %
G = 100 0.15 0.30 0.10 0.15 0.15 0.30 %
G = 1000 0.40 0.70 0.35 0.50 0.40 0.70 %
Nonlinearity VOUT = −10 V to +10 V
G = 1–1000 RL = 10 kΩ 10 40 10 40 10 40 ppm
G = 1–100 RL = 2 kΩ 10 95 10 95 10 95 ppm
Gain vs. Temperature
G = 1 10 10 10 ppm/°C
Gain >12 −50 −50 −50 ppm/°C
VOLTAGE OFFSET (Total RTI Error = VOSI + VOSO/G)
Input Offset, VOSI VS = ±5 V
to ± 15 V
30 125 15 50 30 125 μV
Overtemperature VS = ±5 V
to ± 15 V
185 85 225 μV
Average TC VS = ±5 V
to ± 15 V
0.3 1.0 0.1 0.6 0.3 1.0 μV/°C
Output Offset, VOSO VS = ±15 V 400 1000 200 500 400 1000 μV
VS = ± 5 V 1500 750 1500 μV
Overtemperature VS = ±5 V
to ± 15 V
2000 1000 2000 μV
Average TC VS = ±5 V
to ± 15 V
5.0 15 2.5 7.0 5.0 15 μV/°C
Offset Referred to the
Input vs. Supply (PSR) VS = ±2.3 V
to ±18 V
G = 1 80 100 80 100 80 100 dB
G = 10 95 120 100 120 95 120 dB
G = 100 110 140 120 140 110 140 dB
G = 1000 110 140 120 140 110 140 dB
INPUT CURRENT
Input Bias Current 0.5 2.0 0.5 1.0 0.5 2 nA
Overtemperature 2.5 1.5 4 nA
Average TC 3.0 3.0 8.0 pA/°C
Input Offset Current 0.3 1.0 0.3 0.5 0.3 1.0 nA
Overtemperature 1.5 0.75 2.0 nA
Average TC 1.5 1.5 8.0 pA/°C
INPUT
Input Impedance
Differential 10||2 10||2 10||2 GΩ_pF
Common-Mode 10||2 10||2 10||2 GΩ_pF
Input Voltage Range3 VS = ±2.3 V
to ±5 V
−VS + 1.9 +VS − 1.2 −VS + 1.9 +VS − 1.2 −VS + 1.9 +VS − 1.2 V
Overtemperature −VS + 2.1 +VS − 1.3 −VS + 2.1 +VS − 1.3 −VS + 2.1 +VS − 1.3 V
VS = ± 5 V
to ±18 V
−VS + 1.9 +VS − 1.4 −VS + 1.9 +VS − 1.4 −VS + 1.9 +VS − 1.4 V
Overtemperature −VS + 2.1 +VS − 1.4 −VS + 2.1 +VS + 2.1 −VS + 2.3 +VS − 1.4 V
AD620
Rev. H | Page 4 of 20
AD620A AD620B AD620S1
Parameter Conditions Min Typ Max Min Typ Max Min Typ Max Unit
Common-Mode Rejection
Ratio DC to 60 Hz with
1 kΩ Source Imbalance VCM = 0 V to ± 10 V
G = 1 73 90 80 90 73 90 dB
G = 10 93 110 100 110 93 110 dB
G = 100 110 130 120 130 110 130 dB
G = 1000 110 130 120 130 110 130 dB
OUTPUT
Output Swing RL = 10 kΩ
VS = ±2.3 V
to ± 5 V
−VS +
1.1
+VS − 1.2 −VS + 1.1 +VS − 1.2 −VS + 1.1 +VS − 1.2 V
Overtemperature −VS + 1.4 +VS − 1.3 −VS + 1.4 +VS − 1.3 −VS + 1.6 +VS − 1.3 V
VS = ±5 V
to ± 18 V
−VS + 1.2 +VS − 1.4 −VS + 1.2 +VS − 1.4 −VS + 1.2 +VS − 1.4 V
Overtemperature −VS + 1.6 +VS – 1.5 −VS + 1.6 +VS – 1.5 –VS + 2.3 +VS – 1.5 V
Short Circuit Current ±18 ±18 ±18 mA
DYNAMIC RESPONSE
Small Signal –3 dB Bandwidth
G = 1 1000 1000 1000 kHz
G = 10 800 800 800 kHz
G = 100 120 120 120 kHz
G = 1000 12 12 12 kHz
Slew Rate 0.75 1.2 0.75 1.2 0.75 1.2 V/μs
Settling Time to 0.01% 10 V Step
G = 1–100 15 15 15 μs
G = 1000 150 150 150 μs
NOISE
Voltage Noise, 1 kHz Total RTI Noise (e2 ) (e /G)2 = ni + no
Input, Voltage Noise, eni 9 13 9 13 9 13 nV/√Hz
Output, Voltage Noise, eno 72 100 72 100 72 100 nV/√Hz
RTI, 0.1 Hz to 10 Hz
G = 1 3.0 3.0 6.0 3.0 6.0 μV p-p
G = 10 0.55 0.55 0.8 0.55 0.8 μV p-p
G = 100–1000 0.28 0.28 0.4 0.28 0.4 μV p-p
Current Noise f = 1 kHz 100 100 100 fA/√Hz
0.1 Hz to 10 Hz 10 10 10 pA p-p
REFERENCE INPUT
RIN 20 20 20 kΩ
IIN VIN+, VREF = 0 50 60 50 60 50 60 μA
Voltage Range −VS + 1.6 +VS − 1.6 −VS + 1.6 +VS − 1.6 −VS + 1.6 +VS − 1.6 V
Gain to Output 1 ± 0.0001 1 ± 0.0001 1 ± 0.0001
POWER SUPPLY
Operating Range4 ±2.3 ±18 ±2.3 ±18 ±2.3 ±18 V
Quiescent Current VS = ±2.3 V
to ±18 V
0.9 1.3 0.9 1.3 0.9 1.3 mA
Overtemperature 1.1 1.6 1.1 1.6 1.1 1.6 mA
TEMPERATURE RANGE
For Specified Performance −40 to +85 −40 to +85 −55 to +125 °C
1 See Analog Devices military data sheet for 883B tested specifications.
2 Does not include effects of external resistor RG.
3 One input grounded. G = 1.
4 This is defined as the same supply range that is used to specify PSR.
AD620
Rev. H | Page 5 of 20
ABSOLUTE MAXIMUM RATINGS
Table 3.
Parameter Rating
Supply Voltage ±18 V
Internal Power Dissipation1 650 mW
Input Voltage (Common-Mode) ±VS
Differential Input Voltage 25 V
Output Short-Circuit Duration Indefinite
Storage Temperature Range (Q) −65°C to +150°C
Storage Temperature Range (N, R) −65°C to +125°C
Operating Temperature Range
AD620 (A, B) −40°C to +85°C
AD620 (S) −55°C to +125°C
Lead Temperature Range
(Soldering 10 seconds) 300°C
1 Specification is for device in free air:
8-Lead Plastic Package: θJA = 95°C
8-Lead CERDIP Package: θJA = 110°C
8-Lead SOIC Package: θJA = 155°C
Stresses above those listed under Absolute Maximum Ratings
may cause permanent damage to the device. This is a stress
rating only; functional operation of the device at these or any
other condition s above those indicated in the operational
section of this specification is not implied. Exposure to absolute
maximum rating conditions for extended periods may affect
device reliability.
ESD CAUTION
AD620
Rev. H | Page 6 of 20
TYPICAL PERFORMANCE CHARACTERISTICS
(@ 25°C, VS = ±15 V, RL = 2 kΩ, unless otherwise noted.)
INPUT OFFSET VOLTAGE (μV)
20
30
40
50
–40 0 40 80
PERCENTAGE OF UNITS
–80
SAMPLE SIZE = 360
10
0
00775-0-005
Figure 3. Typical Distribution of Input Offset Voltage
INPUT BIAS CURRENT (pA)
0
10
20
30
40
50
–600 0 600
PERCENTAGE OF UNITS
–1200 1200
SAMPLE SIZE = 850
00775-0-006
Figure 4. Typical Distribution of Input Bias Current
10
20
30
40
50
–200 0 200 400
INPUT OFFSET CURRENT (pA)
PERCENTAGE OF UNITS
–400
0
SAMPLE SIZE = 850 00775-0-007
Figure 5. Typical Distribution of Input Offset Current
TEMPERATURE (°C)
INPUT BIAS CURRENT (nA)
+IB
–IB
2.0
–2.0
175
–1.0
–1.5
–75
–0.5
0
0.5
1.0
1.5
–25 25 75 125
00775-0-008
Figure 6. Input Bias Current vs. Temperature
CHANGE IN OFFSET VOLTAGE (μV)
1.5
0.5
WARM-UP TIME (Minutes)
2.0
0
0 1
1.0
2 3 4 5
00775-0-009
Figure 7. Change in Input Offset Voltage vs. Warm-Up Time
FREQUENCY (Hz)
1000
1
1 100k
100
10
100 1k 10k
VOLTAGE NOISE (nV/ Hz)
GAIN = 1
GAIN = 10
10
GAIN = 100, 1,000
GAIN = 1000
BW LIMIT
00775-0-010
Figure 8. Voltage Noise Spectral Density vs. Frequency (G = 1−1000)
AD620
Rev. H | Page 7 of 20
FREQUENCY (Hz)
1000
100
10
1 10 100 1000
CURRENT NOISE (fA/ Hz)
00775-0-011
Figure 9. Current Noise Spectral Density vs. Frequency
RTI NOISE (2.0μV/DIV)
TIME (1 SEC/DIV)
00775-0-012
Figure 10. 0.1 Hz to 10 Hz RTI Voltage Noise (G = 1)
RTI NOISE (0.1μV/DIV)
TIME (1 SEC/DIV)
00775-0-013
Figure 11. 0.1 Hz to 10 Hz RTI Voltage Noise (G = 1000)
00775-0-014
Figure 12. 0.1 Hz to 10 Hz Current Noise, 5 pA/Div
100
1000
AD620A
FET INPUT
IN-AMP
SOURCE RESISTANCE (Ω)
TOTAL DRIFT FROM 25°C TO 85°C, RTI (μV)
100,000
10
1k 10M
10,000
10k 100k 1M
00775-0-015
Figure 13. Total Drift vs. Source Resistance
FREQUENCY (Hz)
CMR (dB)
160
0
1M
80
40
1
60
0.1
140
100
120
10 100 1k 10k 100k
G = 1000
G = 100
G = 10
G = 1
20
00775-0-016
Figure 14. Typical CMR vs. Frequency, RTI, Zero to 1 kΩ Source Imbalance
AD620
Rev. H | Page 8 of 20
FREQUENCY (Hz)
PSR (dB)
160
1M
80
40
1
60
0.1
140
100
120
10 100 1k 10k 100k
20
G = 1000
G = 100
G = 10
G = 1
180
00775-0-017
Figure 15. Positive PSR vs. Frequency, RTI (G = 1−1000)
FREQUENCY (Hz)
PSR (dB)
160
1M
80
40
1
60
0.1
140
100
120
10 100 1k 10k 100k
20
180
G = 10
G = 100
G = 1
G = 1000
00775-0-018
Figure 16. Negative PSR vs. Frequency, RTI (G = 1−1000)
1000
100 10M
100
1
1k
10
10k 100k 1M
FREQUENCY (Hz)
GAIN (V/V)
0.1
00775-0-019
Figure 17. Gain vs. Frequency
OUTPUT VOLTAGE (V p-p)
FREQUENCY (Hz)
35
0
1M
15
5
10k
10
1k
30
20
25
100k
G = 10, 100, 1000
G = 1
G = 1000 G = 100
BW LIMIT
00775-0-020
Figure 18. Large Signal Frequency Response
INPUT VOLTAGE LIMIT (V)
(REFERRED TO SUPPLY VOLTAGES) 20
+1.0
+0.5
0 5
+1.5
–1.5
–1.0
–0.5
10 15
SUPPLY VOLTAGE ± Volts
+VS –0.0
–VS +0.0
00775-0-021
Figure 19. Input Voltage Range vs. Supply Voltage, G = 1
20
+1.0
+0.5
0 5
+1.5
–1.5
–1.0
–0.5
10 15
SUPPLY VOLTAGE ± Volts
RL = 10kΩ
RL = 2kΩ
RL = 10kΩ
OUTPUT VOLTAGE SWING (V)
(REFERRED TO SUPPLY VOLTAGES)
RL = 2kΩ
+VS
–VS
00775-0-022
–0.0
+0.0
Figure 20. Output Voltage Swing vs. Supply Voltage, G = 10
AD620
Rev. H | Page 9 of 20
OUTPUT VOLTAGE SWING (V p-p)
LOAD RESISTANCE (Ω)
30
0
0 10k
20
10
100 1k
VS = ±15V
G = 10
00775-0-023
Figure 21. Output Voltage Swing vs. Load Resistance
. . . . . . . . . . . . . . . . . . . . . . . . . . . . . . . . . . . . . . . .
. . . . . . . . . . . . . . . . . . . . . . . . . . . . . . . . . . . . . . . .
00775-0-024
Figure 22. Large Signal Pulse Response and Settling Time
G = 1 (0.5 mV = 0.01%)
. . . . . . . . . . . . . . . . . . . . . . . . . . . . . . . . . . . . . . . .
. . . . . . . . . . . . . . . . . . . . . . . . . . . . . . . . . . . . . . . . 00775-0-025
Figure 23. Small Signal Response, G = 1, RL = 2 kΩ, CL = 100 pF
. . . . . . . . . . . . . . . . . . . . . . . . . . . . . . . . . . . . . . . .
. . . . . . . . . . . . . . . . . . . . . . . . . . . . . . . . . . . . . . . .
00775-0-026
Figure 24. Large Signal Response and Settling Time, G = 10 (0.5 mV = 0.01%)
. . . . . . . . . . . . . . . . . . . . . . . . . . . . . . . . . . . . . . . .
. . . . . . . . . . . . . . . . . . . . . . . . . . . . . . . . . . . . . . . .
00775-0-027
Figure 25. Small Signal Response, G = 10, RL = 2 kΩ, CL = 100 pF
. . . . . . . . . . . . . . . . . . . . . . . . . . . . . . . . . . . . . . . .
. . . . . . . . . . . . . . . . . . . . . . . . . . . . . . . . . . . . . . . .
00775-0-030
Figure 26. Large Signal Response and Settling Time, G = 100 (0.5 mV = 0.01%)
AD620
Rev. H | Page 10 of 20
. . . . . . . . . . . . . . . . . . . . . . . . . . . . . . . . . . . . . . . .
. . . . . . . . . . . . . . . . . . . . . . . . . . . . . . . . . . . . . . . .
00775-0-029
Figure 27. Small Signal Pulse Response, G = 100, RL = 2 kΩ, CL = 100 pF
. . . . . . . . . . . . . . . . . . . . . . . . . . . . . . . . . . . . . . . .
. . . . . . . . . . . . . . . . . . . . . . . . . . . . . . . . . . . . . . . .
00775-0-030
Figure 28. Large Signal Response and Settling Time,
G = 1000 (0.5 mV = 0.01% )
. . . . . . . . . . . . . . . . . . . . . . . . . . . . . . . . . . . . . . . .
. . . . . . . . . . . . . . . . . . . . . . . . . . . . . . . . . . . . . . . .
00775-0-031
Figure 29. Small Signal Pulse Response, G = 1000, RL = 2 kΩ, CL = 100 pF
OUTPUT STEP SIZE (V)
SETTLING TIME (μs)
TO 0.01%
TO 0.1%
20
0
0 2
15
5
5
10
10 15 0
00775-0-032
Figure 30. Settling Time vs. Step Size (G = 1)
GAIN
SETTLING TIME (μs)
1000
1
1 1000
100
10
10 100
00775-0-033
Figure 31. Settling Time to 0.01% vs. Gain, for a 10 V Step
. . . . . . . . . . . . . . . . . . . . . . . . . . . . . . . . . . . . . . . .
. . . . . . . . . . . . . . . . . . . . . . . . . . . . . . . . . . . . . . . .
00775-0-034
Figure 32. Gain Nonlinearity, G = 1, RL = 10 kΩ (10 μV = 1 ppm)
AD620
Rev. H | Page 11 of 20
. . . . . . . . . . . . . . . . . . . . . . . . . . . . . . . . . . . . . . . .
. . . . . . . . . . . . . . . . . . . . . . . . . . . . . . . . . . . . . . . .
00775-0-035
Figure 33. Gain Nonlinearity, G = 100, RL = 10 kΩ
(100 μV = 10 ppm)
. . . . . . . . . . . . . . . . . . . . . . . . . . . . . . . . . . . . . . . .
. . . . . . . . . . . . . . . . . . . . . . . . . . . . . . . . . . . . . . . .
00775-0-036
Figure 34. Gain Nonlinearity, G = 1000, RL = 10 kΩ
(1 mV = 100 ppm)
AD620
VOUT
G = 1000 G = 1
49.9Ω
10kΩ *
1kΩ
10T 10kΩ
499Ω
G = 100 G = 10
5.49kΩ
+VS
11kΩ 1kΩ 100Ω
100kΩ
INPUT
10V p-p
–VS
*ALL RESISTORS 1% TOLERANCE
1 7
2
3
8
6
4
5
00775-0-037
Figure 35. Settling Time Test Circuit
AD620
Rev. H | Page 12 of 20
THEORY OF OPERATION
VB
–VS
A1 A2
A3
C2
RG
R1 R2
GAIN
SENSE
GAIN
SENSE
10kΩ
10kΩ
I1 I2
10kΩ
REF
10kΩ
+IN
– IN R4
400Ω
OUTPUT
C1
Q1 Q2
00775-0-038
R3
400Ω
+VS +VS
+VS
20μA 20μA
Figure 36. Simplified Schematic of AD620
The AD620 is a monolithic instrumentation amplifier based on
a modification of the classic three op amp approach. Absolute
value trimming allows the user to program gain accurately
(to 0.15% at G = 100) with only one resistor. Monolithic
construction and laser wafer trimming allow the tight matching
and tracking of circuit components, thus ensuring the high level
of performance inherent in this circuit.
The input transistors Q1 and Q2 provide a single differentialpair
bipolar input for high precision (Figure 36), yet offer 10×
lower input bias current thanks to Superϐeta processing.
Feedback through the Q1-A1-R1 loop and the Q2-A2-R2 loop
maintains constant collector current of the input devices Q1
and Q2, thereby impressing the input voltage across the external
gain setting resistor RG. This creates a differential gain from the
inputs to the A1/A2 outputs given by G = (R1 + R2)/RG + 1. The
unity-gain subtractor, A3, removes any common-mode signal,
yielding a single-ended output referred to the REF pin potential.
The value of RG also determines the transconductance of the
preamp stage. As RG is reduced for larger gains, the
transconductance increases asymptotically to that of the input
transistors. This has three important advantages: (a) Open-loop
gain is boosted for increasing programmed gain, thus reducing
gain related errors. (b) The gain-bandwidth product
(determined by C1 and C2 and the preamp transconductance)
increases with programmed gain, thus optimizing frequency
response. (c) The input voltage noise is reduced to a value of
9 nV/√Hz, determined mainly by the collector current and base
resistance of the input devices.
The internal gain resistors, R1 and R2, are trimmed to an
absolute value of 24.7 kΩ, allowing the gain to be programmed
accurately with a single external resistor.
The gain equation is then
1
49.4 +
Ω
=
RG
k
G
1
49.4
−
Ω
=
G
k
RG
Make vs. Buy: a Typical Bridge Application Error Budget
The AD620 offers improved performance over “homebrew”
three op amp IA designs, along with smaller size, fewer
components, and 10× lower supply current. In the typical
application, shown in Figure 37, a gain of 100 is required to
amplify a bridge output of 20 mV full-scale over the industrial
temperature range of −40°C to +85°C. Table 4 shows how to
calculate the effect various error sources have on circuit
accuracy.
AD620
Rev. H | Page 13 of 20
Regardless of the system in which it is being used, the AD620
provides greater accuracy at low power and price. In simple
systems, absolute accuracy and drift errors are by far the most
significant contributors to error. In more complex systems
with an intelligent processor, an autogain/autozero cycle
removes all absolute accuracy and drift errors, leaving only the
resolution errors of gain, nonlinearity, and noise, thus allowing
full 14-bit accuracy.
Note that for the homebrew circuit, the OP07 specifications for
input voltage offset and noise have been multiplied by √2. This
is because a three op amp type in-amp has two op amps at its
inputs, both contributing to the overall input error.
R = 350Ω
10V
PRECISION BRIDGE TRANSDUCER
R = 350Ω R = 350Ω
R = 350Ω
00775-0-039
AD620A MONOLITHIC
INSTRUMENTATION
AMPLIFIER, G = 100
SUPPLY CURRENT = 1.3mA MAX
AD620A RG
499Ω
REFERENCE
00775-0-040
Figure 37. Make vs. Buy
"HOMEBREW" IN-AMP, G = 100
*0.02% RESISTOR MATCH, 3ppm/°C TRACKING
**DISCRETE 1% RESISTOR, 100ppm/°C TRACKING
SUPPLY CURRENT = 15mA MAX
100Ω **
10kΩ *
10kΩ **
10kΩ *
10kΩ *
10kΩ **
10kΩ*
OP07D
OP07D
OP07D
00775-0-041
Table 4. Make vs. Buy Error Budget
Error, ppm of Full Scale
Error Source AD620 Circuit Calculation “Homebrew” Circuit Calculation AD620 Homebrew
ABSOLUTE ACCURACY at TA = 25°C
Input Offset Voltage, μV 125 μV/20 mV (150 μV × √2)/20 mV 6,250 10,607
Output Offset Voltage, μV 1000 μV/100 mV/20 mV ((150 μV × 2)/100)/20 mV 500 150
Input Offset Current, nA 2 nA ×350 Ω/20 mV (6 nA ×350 Ω)/20 mV 18 53
CMR, dB 110 dB(3.16 ppm) ×5 V/20 mV (0.02% Match × 5 V)/20 mV/100 791 500
Total Absolute Error 7,559 11,310
DRIFT TO 85°C
Gain Drift, ppm/°C (50 ppm + 10 ppm) ×60°C 100 ppm/°C Track × 60°C 3,600 6,000
Input Offset Voltage Drift, μV/°C 1 μV/°C × 60°C/20 mV (2.5 μV/°C × √2 × 60°C)/20 mV 3,000 10,607
Output Offset Voltage Drift, μV/°C 15 μV/°C × 60°C/100 mV/20 mV (2.5 μV/°C × 2 × 60°C)/100 mV/20 mV 450 150
Total Drift Error 7,050 16,757
RESOLUTION
Gain Nonlinearity, ppm of Full Scale 40 ppm 40 ppm 40 40
Typ 0.1 Hz to 10 Hz Voltage Noise, μV p-p 0.28 μV p-p/20 mV (0.38 μV p-p × √2)/20 mV 14 27
Total Resolution Error 54 67
Grand Total Error 14,663 28,134
G = 100, VS = ±15 V.
(All errors are min/max and referred to input.)
AD620
Rev. H | Page 14 of 20
3kΩ
5V
DIGITAL
DATA
OUTPUT
ADC
REF
IN
AGND
20kΩ
10kΩ
20kΩ
G = 100 AD620B
1.7mA 0.10mA 0.6mA
MAX
499Ω
3kΩ
3kΩ 3kΩ
2
1
8
3 7
6
5
4
1.3mA
MAX
AD705
00775-0-042
Figure 38. A Pressure Monitor Circuit that Operates on a 5 V Single Supply
Pressure Measurement
Although useful in many bridge applications, such as weigh
scales, the AD620 is especially suitable for higher resistance
pressure sensors powered at lower voltages where small size and
low power become more significant.
Figure 38 shows a 3 kΩ pressure transducer bridge powered
from 5 V. In such a circuit, the bridge consumes only 1.7 mA.
Adding the AD620 and a buffered voltage divider allows the
signal to be conditioned for only 3.8 mA of total supply current.
Small size and low cost make the AD620 especially attractive for
voltage output pressure transducers. Since it delivers low noise
and drift, it also serves applications such as diagnostic
noninvasive blood pressure measurement.
Medical ECG
The low current noise of the AD620 allows its use in ECG
monitors (Figure 39) where high source resistances of 1 MΩ or
higher are not uncommon. The AD620’s low power, low supply
voltage requirements, and space-saving 8-lead mini-DIP and
SOIC package offerings make it an excellent choice for batterypowered
data recorders.
Furthermore, the low bias currents and low current noise,
coupled with the low voltage noise of the AD620, improve the
dynamic range for better performance.
The value of capacitor C1 is chosen to maintain stability of
the right leg drive loop. Proper safeguards, such as isolation,
must be added to this circuit to protect the patient from
possible harm.
G = 7
AD620A
0.03Hz
HIGHPASS
FILTER
OUTPUT
1V/mV
+3V
–3V
RG
8.25kΩ
24.9kΩ
24.9kΩ
AD705J
G = 143
C1
1MΩ
R4
10kΩ
R1 R3
R2
OUTPUT
AMPLIFIER
PATIENT/CIRCUIT
PROTECTION/ISOLATION
00775-0-043
Figure 39. A Medical ECG Monitor Circuit
AD620
Rev. H | Page 15 of 20
Precision V-I Converter
The AD620, along with another op amp and two resistors,
makes a precision current source (Figure 40). The op amp
buffers the reference terminal to maintain good CMR. The
output voltage, VX, of the AD620 appears across R1, which
converts it to a current. This current, less only the input bias
current of the op amp, then flows out to the load.
RG AD620
–VS
VIN+
VIN–
LOAD
R1
IL
Vx I L = R1
= IN+ [(V ) – (V IN – )] G
R1
6
5
+ VX –
2 4
1
8
3 7
+VS
AD705
00775-0-044
Figure 40. Precision Voltage-to-Current Converter (Operates on 1.8 mA, ±3 V)
GAIN SELECTION
The AD620 gain is resistor-programmed by RG, or more
precisely, by whatever impedance appears between Pins 1 and 8.
The AD620 is designed to offer accurate gains using 0.1% to 1%
resistors. Table 5 shows required values of RG for various gains.
Note that for G = 1, the RG pins are unconnected (RG = ∞). For
any arbitrary gain, RG can be calculated by using the formula:
1
49.4
−
Ω
=
G
k
RG
To minimize gain error, avoid high parasitic resistance in series
with RG; to minimize gain drift, RG should have a low TC—less
than 10 ppm/°C—for the best performance.
Table 5. Required Values of Gain Resistors
1% Std Table
Value of RG(Ω)
Calculated
Gain
0.1% Std Table
Value of RG(Ω )
Calculated
Gain
49.9 k 1.990 49.3 k 2.002
12.4 k 4.984 12.4 k 4.984
5.49 k 9.998 5.49 k 9.998
2.61 k 19.93 2.61 k 19.93
1.00 k 50.40 1.01 k 49.91
499 100.0 499 100.0
249 199.4 249 199.4
100 495.0 98.8 501.0
49.9 991.0 49.3 1,003.0
INPUT AND OUTPUT OFFSET VOLTAGE
The low errors of the AD620 are attributed to two sources,
input and output errors. The output error is divided by G when
referred to the input. In practice, the input errors dominate at
high gains, and the output errors dominate at low gains. The
total VOS for a given gain is calculated as
Total Error RTI = input error + (output error/G)
Total Error RTO = (input error × G) + output error
REFERENCE TERMINAL
The reference terminal potential defines the zero output voltage
and is especially useful when the load does not share a precise
ground with the rest of the system. It provides a direct means of
injecting a precise offset to the output, with an allowable range
of 2 V within the supply voltages. Parasitic resistance should be
kept to a minimum for optimum CMR.
INPUT PROTECTION
The AD620 safely withstands an input current of ±60 mA for
several hours at room temperature. This is true for all gains and
power on and off, which is useful if the signal source and
amplifier are powered separately. For longer time periods, the
input current should not exceed 6 mA.
For input voltages beyond the supplies, a protection resistor
should be placed in series with each input to limit the current to
6 mA. These can be the same resistors as those used in the RFI
filter. High values of resistance can impact the noise and AC
CMRR performance of the system. Low leakage diodes (such as
the BAV199) can be placed at the inputs to reduce the required
protection resistance.
AD620
R
REF
R
+SUPPLY
–SUPPLY
VOUT
+IN
–IN
00775-0-052
Figure 41. Diode Protection for Voltages Beyond Supply
RF INTERFERENCE
All instrumentation amplifiers rectify small out of band signals.
The disturbance may appear as a small dc voltage offset. High
frequency signals can be filtered with a low pass R-C network
placed at the input of the instrumentation amplifier. Figure 42
demonstrates such a configuration. The filter limits the input
AD620
Rev. H | Page 16 of 20
signal according to the following relationship:
2 (2 )
1
D C
DIFF R C C
FilterFreq
π +
=
C
CM RC
FilterFreq
π
=
2
1
where CD ≥10CC.
CD affects the difference signal. CC affects the common-mode
signal. Any mismatch in R × CC degrades the AD620 CMRR. To
avoid inadvertently reducing CMRR-bandwidth performance,
make sure that CC is at least one magnitude smaller than CD.
The effect of mismatched CCs is reduced with a larger CD:CC
ratio.
499Ω AD620
+
–
VOUT
R
R
CC
CD
CC +IN
–IN REF
–15V
0.1μ F 10μ F
+15V
0.1μ F 10μ F
00775-0-045
Figure 42. Circuit to Attenuate RF Interference
COMMON-MODE REJECTION
Instrumentation amplifiers, such as the AD620, offer high
CMR, which is a measure of the change in output voltage when
both inputs are changed by equal amounts. These specifications
are usually given for a full-range input voltage change and a
specified source imbalance.
For optimal CMR, the reference terminal should be tied to a
low impedance point, and differences in capacitance and
resistance should be kept to a minimum between the two
inputs. In many applications, shielded cables are used to
minimize noise; for best CMR over frequency, the shield
should be properly driven. Figure 43 and Figure 44 show active
data guards that are configured to improve ac common-mode
rejections by “bootstrapping” the capacitances of input cable
shields, thus minimizing the capacitance mismatch between the
inputs.
REFERENCE
VOUT AD620
100Ω
100Ω
– INPUT
+ INPUT
AD648
RG
–VS
+VS
–VS
00775-0-046
Figure 43. Differential Shield Driver
100Ω
– INPUT
+ INPUT
REFERENCE
VOUT AD620
–VS
+VS
2
RG
2
RG AD548
00775-0-047
Figure 44. Common-Mode Shield Driver
GROUNDING
Since the AD620 output voltage is developed with respect to the
potential on the reference terminal, it can solve many
grounding problems by simply tying the REF pin to the
appropriate “local ground.”
To isolate low level analog signals from a noisy digital
environment, many data-acquisition components have separate
analog and digital ground pins (Figure 45). It would be
convenient to use a single ground line; however, current
through ground wires and PC runs of the circuit card can cause
hundreds of millivolts of error. Therefore, separate ground
returns should be provided to minimize the current flow from
the sensitive points to the system ground. These ground returns
must be tied together at some point, usually best at the ADC
package shown in Figure 45.
DIGITAL P.S.
C +5V
ANALOG P.S.
+15V C –15V
AD574A DIGITAL
DATA
OUTPUT
+
1μF
AD620
0.1μF
AD585
S/H ADC
0.1μF
1μF 1μF
00775-0-048
Figure 45. Basic Grounding Practice
AD620
Rev. H | Page 17 of 20
GROUND RETURNS FOR INPUT BIAS CURRENTS
VOUT
– INPUT
+ INPUT
RG
LOAD
TO POWER
SUPPLY
GROUND
REFERENCE
+VS
–VS
AD620
00775-0-050
Input bias currents are those currents necessary to bias the
input transistors of an amplifier. There must be a direct return
path for these currents. Therefore, when amplifying “floating”
input sources, such as transformers or ac-coupled sources, there
must be a dc path from each input to ground, as shown in
Figure 46, Figure 47, and Figure 48. Refer to A Designer’s Guide
to Instrumentation Amplifiers (free from Analog Devices) for
more information regarding in-amp applications.
AD620 VOUT
– INPUT
RG
TO POWER
SUPPLY
GROUND
+ INPUT REFERENCE
+VS
–VS
LOAD
00775-0-049
Figure 47. Ground Returns for Bias Currents with Thermocouple Inputs
100kΩ
AD620 VOUT
– INPUT
+ INPUT
RG
LOAD
TO POWER
SUPPLY
GROUND
REFERENCE
100kΩ –VS
+VS
00775-0-051
Figure 46. Ground Returns for Bias Currents with Transformer-Coupled Inputs
Figure 48. Ground Returns for Bias Currents with AC-Coupled Inputs
AD620
Rev. H | Page 18 of 20
AD620ACHIPS INFORMATION
Die size: 1803 μm × 3175 μm
Die thickness: 483 μm
Bond Pad Metal: 1% Copper Doped Aluminum
To minimize gain errors introduced by the bond wires, use Kelvin connections between the chip and the gain resistor, RG, by connecting
Pad 1A and Pad 1B in parallel to one end of RG and Pad 8A and Pad 8B in parallel to the other end of RG. For unity gain applications
where RG is not required, Pad 1A and Pad 1B must be bonded together as well as the Pad 8A and Pad 8B.
1A
1B
2
3
4 5
6
7
8A
8B
LOGO
00775-0-053
Figure 49. Bond Pad Diagram
Table 6. Bond Pad Information
Pad Coordinates1
Pad No. Mnemonic X (μm) Y (μm)
1A RG −623 +1424
1B RG −789 +628
2 −IN −790 +453
3 +IN −790 −294
4 −VS −788 −1419
5 REF +570 −1429
6 OUTPUT +693 −1254
7 +VS +693 +139
8A RG +505 +1423
8B RG +693 +372
1 The pad coordinates indicate the center of each pad, referenced to the center of the die. The die orientation is indicated by the logo, as shown in Figure 49.
AD620
Rev. H | Page 19 of 20
OUTLINE DIMENSIONS
COMPLIANT TO JEDEC STANDARDS MS-001
CONTROLLING DIMENSIONS ARE IN INCHES; MILLIMETER DIMENSIONS
(IN PARENTHESES) ARE ROUNDED-OFF INCH EQUIVALENTS FOR
REFERENCE ONLY AND ARE NOT APPROPRIATE FOR USE IN DESIGN.
CORNER LEADS MAY BE CONFIGURED AS WHOLE OR HALF LEADS.
070606-A
0.022 (0.56)
0.018 (0.46)
0.014 (0.36)
SEATING
PLANE
0.015
(0.38)
MIN
0.210 (5.33)
MAX
0.150 (3.81)
0.130 (3.30)
0.115 (2.92)
0.070 (1.78)
0.060 (1.52)
0.045 (1.14)
8
1 4
5 0.280 (7.11)
0.250 (6.35)
0.240 (6.10)
0.100 (2.54)
BSC
0.400 (10.16)
0.365 (9.27)
0.355 (9.02)
0.060 (1.52)
MAX
0.430 (10.92)
MAX
0.014 (0.36)
0.010 (0.25)
0.008 (0.20)
0.325 (8.26)
0.310 (7.87)
0.300 (7.62)
0.195 (4.95)
0.130 (3.30)
0.115 (2.92)
0.015 (0.38)
GAUGE
PLANE
0.005 (0.13)
MIN
Figure 50. 8-Lead Plastic Dual In-Line Package [PDIP]
Narrow Body (N-8).
Dimensions shown in inches and (millimeters)
CONTROLLING DIMENSIONS ARE IN INCHES; MILLIMETER DIMENSIONS
(IN PARENTHESES) ARE ROUNDED-OFF INCH EQUIVALENTS FOR
REFERENCE ONLY AND ARE NOT APPROPRIATE FOR USE IN DESIGN.
0.310 (7.87)
0.220 (5.59)
0.005 (0.13)
MIN
0.055 (1.40)
MAX
0.100 (2.54) BSC
15°
0°
0.320 (8.13)
0.290 (7.37)
0.015 (0.38)
SEATING 0.008 (0.20)
PLANE
0.200 (5.08)
MAX
0.405 (10.29) MAX
0.150 (3.81)
MIN
0.200 (5.08)
0.125 (3.18)
0.023 (0.58)
0.014 (0.36) 0.070 (1.78)
0.030 (0.76)
0.060 (1.52)
0.015 (0.38)
1 4
8 5
Figure 51. 8-Lead Ceramic Dual In-Line Package [CERDIP]
(Q-8)
Dimensions shown in inches and (millimeters)
CONTROLLING DIMENSIONS ARE IN MILLIMETERS; INCH DIMENSIONS
(IN PARENTHESES) ARE ROUNDED-OFF MILLIMETER EQUIVALENTS FOR
REFERENCE ONLY AND ARE NOT APPROPRIATE FOR USE IN DESIGN.
COMPLIANT TO JEDEC STANDARDS MS-012-AA
012407-A
0.25 (0.0098)
0.17 (0.0067)
1.27 (0.0500)
0.40 (0.0157)
0.50 (0.0196)
0.25 (0.0099)
45°
8°
0°
1.75 (0.0688)
1.35 (0.0532)
SEATING
PLANE
0.25 (0.0098)
0.10 (0.0040)
1 4
8 5
5.00 (0.1968)
4.80 (0.1890)
4.00 (0.1574)
3.80 (0.1497)
1.27 (0.0500)
BSC
6.20 (0.2441)
5.80 (0.2284)
0.51 (0.0201)
0.31 (0.0122)
COPLANARITY
0.10
Figure 52. 8-Lead Standard Small Outline Package [SOIC_N]
Narrow Body (R-8)
Dimensions shown in millimeters and (inches)
AD620
Rev. H | Page 20 of 20
ORDERING GUIDE
Model1 Temperature Range Package Description Package Option
AD620AN −40°C to +85°C 8-Lead PDIP N-8
AD620ANZ −40°C to +85°C 8-Lead PDIP N-8
AD620BN −40°C to +85°C 8-Lead PDIP N-8
AD620BNZ −40°C to +85°C 8-Lead PDIP N-8
AD620AR −40°C to +85°C 8-Lead SOIC_N R-8
AD620ARZ −40°C to +85°C 8-Lead SOIC_N R-8
AD620AR-REEL −40°C to +85°C 8-Lead SOIC_N, 13" Tape and Reel R-8
AD620ARZ-REEL −40°C to +85°C 8-Lead SOIC_N, 13" Tape and Reel R-8
AD620AR-REEL7 −40°C to +85°C 8-Lead SOIC_N, 7" Tape and Reel R-8
AD620ARZ-REEL7 −40°C to +85°C 8-Lead SOIC_N, 7" Tape and Reel R-8
AD620BR −40°C to +85°C 8-Lead SOIC_N R-8
AD620BRZ −40°C to +85°C 8-Lead SOIC_N R-8
AD620BR-REEL −40°C to +85°C 8-Lead SOIC_N, 13" Tape and Reel R-8
AD620BRZ-RL −40°C to +85°C 8-Lead SOIC_N, 13" Tape and Reel R-8
AD620BR-REEL7 −40°C to +85°C 8-Lead SOIC_N, 7" Tape and Reel R-8
AD620BRZ-R7 −40°C to +85°C 8-Lead SOIC_N, 7" Tape and Reel R-8
AD620ACHIPS −40°C to +85°C Die Form
AD620SQ/883B −55°C to +125°C 8-Lead CERDIP Q-8
1 Z = RoHS Compliant Part.
© 2003–2011 Analog Devices, Inc. All rights reserved. Trademarks
and registered trademarks are the property of their respective owners.
C00775–0–7/11(H)
a Compensating the dead time of voltage inverters with the ADMC331 AN331-50
© Analog Devices Inc., August 2000 Page 1 of 18
a
Compensating
the dead time of
voltage inverters
with the ADMC331
AN331-50
a Compensating the dead time of voltage inverters with the ADMC331 AN331-50
© Analog Devices Inc., August 2000 Page 2 of 18
Table of Contents
SUMMARY...................................................................................................................... 3
1 DEAD TIME EFFECTS AND THEIR COMPENSATION .......................................... 3
2 IMPLEMENTATION OF THE FEED FORWARD DEAD TIME COMPENSATION .. 5
2.1 Using the dt_comp routines ...........................................................................................................................5
2.2 Using the dt_comp routine.............................................................................................................................6
2.3 The program code...........................................................................................................................................7
3 EXAMPLE: TESTING THE VALIDITY OF THE FEED FORWARD DEAD TIME
COMPENSATION........................................................................................................... 7
3.1 The construction of an inverter .....................................................................................................................7
3.2 The software program used to test the feed forward dead time compensation.........................................8
3.3 The main include file: main.h ......................................................................................................................12
3.4 The program offset.dsp and its header offset.h..........................................................................................12
3.5 Experimental results.....................................................................................................................................16
4 REFERENCES ....................................................................................................... 18
a Compensating the dead time of voltage inverters with the ADMC331 AN331-50
© Analog Devices Inc., August 2000 Page 3 of 18
Summary
Due to the finite switching time, in order to prevent the appearance of short circuits, the power devices of
an inverter must be commanded introducing a delay between their active times. This delay, called dead
time because in this period no power device is active, introduces small voltage errors, which are sufficient
to produce distorted motor currents, oscillations of the motor torque and therefore even the motor
controllability may be lost [1].
This paper presents one method to compensate the effects of the dead time, the experimental hardware on
which this method was tested and the assembly program associated with it.
1 Dead Time effects and their compensation
Consider a voltage inverter with a motor connected at its output terminals (Figure 1).
d V
T1
T 2
T 3
T 4
T 5
T 6
D1
D2 D4 D6
D3 D5
0 1 ≥ s i
1 v
Figure 1: Voltage source inverter
The effects of the dead time may be examined by considering only the first phase of the inverter. On this
phase it is desired to obtain the reference PWM signal *
1 v presented in Figure 2a. The signals used to
command the power devices are assumed to be active LOW, which means that when they are LOW, the
power devices conduct (Figures 2b and 2c). The output signal obtained at the motor terminal depends on
the sense of the current flowing in this phase:
In the case of the current flowing from inverter to the motor (assumed positive sense), when T2 conducts,
the phase terminal is linked to the GND and the voltage 1 v is 0. During the dead time period, when both
power devices are turned OFF, the current continues to flow into the motor using the reverse recovery
diode D2, so 1 v will continue to be 0. When the upper power device T1 conducts, the phase terminal is
connected to d V and 1 v is equal to d V . During the second half cycle, the phenomenon repeats itself
a Compensating the dead time of voltage inverters with the ADMC331 AN331-50
© Analog Devices Inc., August 2000 Page 4 of 18
symmetrically. The final behaviour of 1 v is presented in Figure 2d. It may be observed that the average
value of 1 v is less than the reference value by an amount determined by the dead time:
d
s
V
T
DT
v = v − ⋅ *
1 1 (1)
DT DT 2 DT 2
s T
*
1 v
T1
T 2
0
1
1
≥ s i
v when
0
1
1
< s i
v when
a)
b)
c)
d)
e)
d V
d V
*
1 T
Figure 2: The influence of the dead time over the output phase voltage
In the case of the current flowing from the motor to the inverter, when T2 conducts, the phase terminal is
linked to the GND and the voltage 1 v is 0. During the dead time period, the current continues to flow from
the motor using the reverse recovery diode D1, so 1 v will become equal to d V . When the upper power
device T1 conducts, the phase terminal is connected to d V and 1 v will continue to be equal to d V . During
the second half, the phenomenon repeats itself symmetrically. The final behaviour of 1 v is presented in
Figure 2e. It may be observed that the average value of 1 v is greater than the reference value by an
amount determined by the dead time:
a Compensating the dead time of voltage inverters with the ADMC331 AN331-50
© Analog Devices Inc., August 2000 Page 5 of 18
d
s
V
T
DT
v = v + ⋅ *
1 1 (2)
Equations (1) and (2) provide the first method to compensate for the dead time: the feed-forward
compensation. In relation of the current sense, the inverter phase will be commanded with a reference
voltage **
1 v such that the voltage 1 v at the inverter terminal will become equal with the reference voltage
*
1 v :
d
s
V
T
DT
v = v + ⋅ *
1
**
1 when i ≥ 0 (3)
d
s
V
T
DT
v = v − ⋅ *
1
**
1 when i < 0 .
These expressions mean that when the phase current is positive, the duty cycle *
1 T correspondent to *
1 v
has to be increased by the dead time and when the phase current is negative, the duty cycle has to be
decreased by the dead time. The only drawback of this method appears when the current changes its sign,
because this moment cannot be foreseen. It is easily seen that when the sign is not correctly applied, an
error of two times the dead time is introduced.
Another method to compensate the dead time is the following: The actual inverter voltages are measured
on every phase. The compensation is done adding to the reference phase voltage *
1 v a term proportional
to the voltage error on that phase:
( 1) ( 1) [ ( ) ( )] 1
*
1
*
1
**
1 v k + = v k + + K ⋅ v k − v k (4)
where:
- ** ( 1)
1 v k + is the voltage which will be commanded on the first inverter phase;
- * ( 1)
1 v k + is the reference voltage which would have been commanded if the dead time
compensation had not been considered;
-K is the gain of the compensator, usually less than or equal to 1;
- * ( )
1 v k is the reference voltage which would have been commanded during the previous PWM
cycle if the dead time compensation had not been considered;
- ( ) 1 v k is the inverter phase voltage measured during the previous PWM cycle.
The drawback of this method is that all the inverter phase voltages have to be measured. It is possible to
measure only two inverter phases if the PWM modulation is space vector type or sinusoidal.
2 Implementation of the feed forward dead time compensation
2.1 Using the dt_comp routines
The routines are developed as an easy-to-use library, which has to be linked to the user’s application. The
library consists of two files. The file “dt_comp.dsp” contains the assembly code of the subroutines. The
block has to be compiled and then linked to an application. The user has to include the header file
dt_comp.h, which provides the function-like calls to the subroutines. The example file in Section 3 will
demonstrate the usage of all the routines.
a Compensating the dead time of voltage inverters with the ADMC331 AN331-50
© Analog Devices Inc., August 2000 Page 6 of 18
Operation Usage
Compute On-times compensating the
dead time
DeadTime_Comp(StatorCurrent_struct, Dutycycles_struct)
Table 1 Implemented routine
The input vector StatorCurrents_struct consists of three elements, the three inverter phase currents.
Because their sum is always zero, only two of them need to be measured. They have to be scaled because
the DSP uses fixed point formats. The scaling factor is 2⋅ Imax , where max I represents the maximum
current which may be placed at the input pin of the A/D converter. The 2 factor is used to prevent
overflows when the currents are used in arithmetical operations.
The vector Dutycycles_struct is an input and also an output: It represents the duty cycles for each phase,
previously computed by the PWM modulator. After the compensation, they represent the duty cycles
effectively commanded to the inverter. Their values have to be between 0 and PWMTM, the number
which controls the PWM switching frequency.
DeadTime_comp represents a macro, which must be introduced into the program code if the dead time
compensation is desired. The format of inputs and outputs are explained in more detail in the next section.
The routines do not require any configuration constants from the main include-file “main.h” that comes
with every application note. For more information about the general structure of the application notes and
including libraries into user applications refer to the Library Documentation File. Section 2.2 shows an
example of usage of this library. In the following sections each routine is explained in detail with the
relevant segments of code which is found in either “dt_comp.h” or “dt_comp.dsp”. For more information
see the comments in those files.
2.2 Using the dt_comp routine
The macro listed in the Table 1 is based on a subroutine called DeadTime_Comp_. It is described in
detail in the next section. The following table gives an overview of what DSP registers are used in this
macro:
Macro Input1 and modified DAG
registers
Output2 Modified core registers
DeadTime_Comp I1 = ^ StatorCurrents_struct;
M1, M2 = 1; L1, L2 = 0;
I2 = ^ Dutycycles_struct; M3 = 0;
N/A AX0, AY0, AY1, MR,
AR
Table2. DSP core registers used in the macro
This macro has to be placed in the main program after the PWM reference duty cycles are computed, but
prior to the program that saves them into the duty cycle registers PWMCHA, PWMCHB, PWMCHC.
1 ^vector stands for ‘address of vector’.
2 N/A: The output values are stored in the output vector in the Data memory. No DSP core register is
used.
a Compensating the dead time of voltage inverters with the ADMC331 AN331-50
© Analog Devices Inc., August 2000 Page 7 of 18
2.3 The program code
The following code contained in the file dt_comp.dsp describes the routine DeadTime_Comp_
mentioned in the previous section.
The routine is organised as a loop managed by the loop counter cntr. At each iteration, one phase current
from the buffer StatorCurrents_struct is tested and the compensation is done function of its sign. In the end, the new
duty cycle number is tested to ensure it is positive and less than the maximum admissible value, PWMTM. The last
instruction saves the number back into the buffer Dutycycles_struct.
DeadTime_Comp_:
AY0 = DM(PWMDT); { dead time normalized }
AY1 = dm(PWMTM);
CNTR = 3;
do dead_loop until ce;
ax0 = DM(I1, M1); { ax0 = Isk, k=1,2,3 }
mr1 = DM(I2, M3); { load Ta, Tb, Tc }
AR = MR1 + AY0;
none = pass ax0; {chek sign of the currents }
IF LT AR = MR1 - AY0;
none = pass AR;
if lt AR = PASS 0; { no negative values admitted}
af = AR - AY1;
if gt ar = pass ay1; { protection against overflows}
dead_loop:
DM(I2, M2) = ar;
rts;
3 Example: Testing the validity of the feed forward dead time
compensation
3.1 The construction of the inverter
The proposed compensation method was implemented on the ADMC331 Processor Board mounted on
an ADMC Connector Board. As inverter power part was used an evaluation platform produced by
International Rectifier, IRPT2056D Driver-Plus Board. It is a three phase 230VAC 3HP board and it
integrates all the processing components needed for a 3 HP motor drive. It is equipped with an
IRPT2056A IGBT power module and an IR2133J driver. The Analog Devices’ ADMC PWM isolation
board linked the Connector Board to the Power Board. This board produces an electric isolation between
the digital part and the inverter power part and also inverts the signals used to drive the power devices
(74HC240). Because the signals used by the driver IR2133J are active LOW and because of the inverting
line driver HC240, the PWM outputs of the ADMC331 are set to be active HIGH. Therefore the jumper
JP51 is in position 1-2.
1 See the ADMC331 Processor Board manual, Motion Control Group, Analog Devices, 1998
a Compensating the dead time of voltage inverters with the ADMC331 AN331-50
© Analog Devices Inc., August 2000 Page 8 of 18
The inverter is driving an induction motor with the following characteristics: .13HP, 230V, 60Hz,
1725rpm, produced by Baldor. Because the power part is supplied with 110V, the maximum frequency
the motor may be run in the constant torque regime is:
c f
3
110 2
60
3
230 2 ⋅
=
⋅
f Hz c 28.7
230
110 60 = ⋅ =
Because the compensation needs the value of the inverter phase currents, two of them were sensed using
current transducers HA 10-NP produced by LEM. They are capable to measure up to 20A and this value
is used to scale down the measured values: I 20A max = . Also, an operational amplifier LM348 is used to
obtain the signal into the range of A/D converter of ADMC331: 0.3V÷3.5V. On the ADMC331 Processor
Board there are 5KHz filters that have an anti-aliasing role.
A block structure of the inverter is presented in Figure 3.
ADMCConnector Board
ADMC331 Processor
Board
IRPT2056D Driver Plus Board
ADMC PWM
Isolation Board
.13HP Induction
Motor
2xHP10-NP
s1 I
s 2 I
Figure 3. Inverter Block structure
3.2 The software program used to test the feed forward dead time compensation
The purpose of this program is to demonstrate the improvement offered by the feed forward dead time
compensation. It reads two motor currents, commands the motor to run at 14Hz, half of the cut frequency
c f and compensates for the dead time.
The file main.dsp contains the root program. The batch file build.bat compiles every file of the project,
links them together and builds the executable file main.exe. It may be applied either within DOS prompt
or clicking on it from Windows Explorer. Main.exe may be run on the Motion Control Debugger.
A brief description of the program will be given in the following:
Start of code - declaring start location in program memory
.MODULE/RAM/SEG=USER_PM1/ABS=0x30 Main_Program;
a Compensating the dead time of voltage inverters with the ADMC331 AN331-50
© Analog Devices Inc., August 2000 Page 9 of 18
Next, the general systems constants and PWM configuration constants (main.h – see the next section) are
included. Also included are the PWM library2, the DAC interface3 and the space vector modulation4 module
definitions. The header file offset.h declares some macros used to measure the offset introduced by the current
transducers and autocal.h declares the macros used to calibrate the ADMC331 A/D converter.
{***************************************************************************************
* Include General System Parameters and Libraries *
***************************************************************************************}
#include ;
#include ;
#include ;
#include ;
#include ;
#include ;
#include ;
#include ;
#include ; { Application Specific Module }
#include ;
#include ;
#include ;
Constants used in this program
{***************************************************************************************
* Constants Defined in the Module *
***************************************************************************************}
.CONST CUT_FREQ = 28; {the cutting frequency of the tested motor}
.CONST Delta = 32768*2*CUT_FREQ/PWM_freq; {the increment of the angle}
.CONST TwoPiOverThree = 0xffff / 3; { Hex equivalent of 2pi/3 }
.CONST ALLOFF = 0x3F; { Used to disable IGBTies into PWMSEG }
Here is where all the vectors for the program are declared. The buffer StatorCurrents_struct represents
the three stator currents. The PWM duty cycles are stored in the buffer Dutycycles_struct and they are initialised
with 0.It may be seen that the variables which identify the current offsets, Is1Offset and Is2Offset are declared
circular because programming becomes easier. The average of the readings is computed on 32bit precision, so
every buffer consists of 2 words.
{***************************************************************************************
* Local Variables Defined in this Module *
***************************************************************************************}
.VAR/DM/RAM/SEG=USER_DM AD_IN; { Volts/Hertz Command (0-1) }
2 see AN331-03: Three-Phase Sine-Wave Generation using the PWM Unit of the ADMC331
3 see AN331-06: Using the Serial Digital to Analog Converter of the ADMC Connector Board
4 see AN331-17: Implementing Space Vector Modulation with the ADMC331
a Compensating the dead time of voltage inverters with the ADMC331 AN331-50
© Analog Devices Inc., August 2000 Page 10 of 18
.INIT AD_IN : 0x3A0A; { Corresponds to 0.906/2 }
.VAR/DM/RAM/SEG=USER_DM Theta; { Current angle }
.INIT Theta : 0x0000;
.VAR/DM/RAM/SEG=USER_DM Vdq_ref[2]; { rotor ref.frame }
.VAR/DM/RAM/CIRC/SEG=USER_DM Valphabeta_ref[2]; { alphabeta frame }
.VAR/RAM/DM/SEG=USER_DM OnTime_struct[1*4];
.INIT OnTime_struct: 0x0000, 0x0000, 0x0000, 0x0000;
.VAR/RAM/DM/SEG=USER_DM Dutycycles_struct[1*3];
.INIT Dutycycles_struct: 0x0000, 0x0000, 0x0000;
.VAR/DM/RAM/SEG=USER_DM VrefA; { Voltage demands }
.VAR/DM/RAM/SEG=USER_DM VrefB;
.VAR/DM/RAM/SEG=USER_DM VrefC;
.INIT VrefA : 0x0000;
.INIT VrefB : 0x0000;
.INIT VrefC : 0x0000;
.VAR/DM/RAM/SEG=USER_DM StatorCurrents_struct[1*3]; { stator currents }
.VAR/DM/RAM/SEG=USER_DM Is1Offset[1];
.VAR/DM/RAM/SEG=USER_DM Is2Offset[1];
When the program begins, the PWM output signals are disabled. Then, the power module is reset and the
PWM block is set up to generate interrupts every 100μsec (see main.h in the next section). There is initialised the
D/A serial converter1 and there is unmasked the IRQ2 interrupt (the interrupt which manages the peripheral
interrupts on ADMC331). The main loop just waits for interrupts.
{********************************************************************************************}
{ Start of program code }
{********************************************************************************************}
Startup:
Write_DM(PWMSEG, ALLOFF); { the IGBTies are disabled }
IR_reset_PIO3; { Reset PowIRTrain Module }
PWM_Init(PWMSYNC_ISR, PWMTRIP_ISR);
DAC_Init; { Initialize the DAC-Module }
IFC = 0x80; { Clear any pending IRQ2 inter. }
ay0 = 0x200; { unmask irq2 interrupts. }
ar = IMASK;
ar = ar or ay0;
1 See ADMC Connector board user’s manual for further details
a Compensating the dead time of voltage inverters with the ADMC331 AN331-50
© Analog Devices Inc., August 2000 Page 11 of 18
IMASK = ar; { IRQ2 ints fully enabled here }
ADC_Init; { ADC Counter will Operate at the DSP CLKOUT Frequency }
AutoCal_Init; { Initialize the Auto Calibration Routine }
Offset_Init; { offset.h }
Main: { Wait for interrupt to occur }
jump Main;
During the PWM_SYNC interrupt there are executed some routines which determine the internal offset of
the A/D converter1, the external offsets introduced by the current transducers and the measurement of the currents.
The successive routines generate three PWM signals of 14Hz obtained applying a continuous space vector
modulation2. The dead time compensation is placed at the end of this block. Finally, the signals that will be provided
to the D/A converter are computed.
{********************************************************************************************}
{ PWM Interrupt Service Routine }
{********************************************************************************************}
PWMSYNC_ISR:
Auto_Calibrate; { autocal.h }
OffsetDetermination(ADC1, ADC2, Is1Offset, Is2Offset); { offset.h }
ReadCurrents(Is1Offset, Is2Offset, StatorCurrents_struct, ADC1, ADC2); { offset.h }
DAC_Pause; { Required only when I1, M1 or L1 is used}
ar = DM (AD_IN );
mr = 0; {Clear mr }
mr1 = dm(Theta); {Preload Theta}
my0 = Delta;
mr = mr + ar*my0 (SS); {Compute new angle & store}
dm(Theta) = mr1;
DM(Vdq_ref )= ar; {Set constant Vdq reference (AD_IN,0)}
ar = pass 0;
DM(Vdq_ref+1)= ar;
refframe_Set_DAG_registers_for_transformations;
refframe_Forward_Park_angle(Vdq_ref,Valphabeta_ref,mr1); {generate Vreference in alpha-beta frame}
SVPWM_Calc_Ontimes(Valphabeta_ref, OnTime_struct); { use SVPWM routines}
SVPWM_Calc_Dutycycles(OnTime_struct, Dutycycles_struct);
DeadTime_Comp(StatorCurrents_struct, Dutycycles_struct);
SVPWM_Update_DutyCycles(Dutycycles_struct);
Dac_Resume;
my0 = DM(Theta); DAC_Put(1, my0); { output on DACs, amplified by multiplication }
mx0 = 0x8;
my0 = DM(Dutycycles_struct ); mr = mx0 * my0 (SS); Dac_Put(2, mr0);
my0 = DM(Dutycycles_struct+1); mr = mx0 * my0 (SS); Dac_Put(3, mr0);
1 See AN331-05: ADC-system on the ADMC331.
2 See AN331-17: Implementing Space Vector Modulation with ADMC331
a Compensating the dead time of voltage inverters with the ADMC331 AN331-50
© Analog Devices Inc., August 2000 Page 12 of 18
AX0 = dm(Dutycycles_struct);
AY0 = Half_PWMTM;
AR = AX0 - AY0;
MY0 = 0x6523; {2/PWMTM=2/1296*2^15/2^6*2^15}
MR = AR * MY0 (SS);
SR = ASHIFT MR1 BY 6 (HI);
SR = SR OR LSHIFT MR0 BY 6 (LO);
DAC_Put(4, SR1);
sr1 = DM(StatorCurrents_struct); sr = ASHIFT sr1 BY 5 (HI); DAC_Put(5,sr1);
sr1 = DM(StatorCurrents_struct+1); sr = ASHIFT sr1 BY 5 (HI); DAC_Put(6, sr1);
SR1 = DM(StatorCurrents_struct+2); sr = ASHIFT sr1 BY 5 (HI); DAC_Put(7, sr1);
DAC_Update;
RTI;
3.3 The main include file: main.h
This file contains the definitions of ADMC331 constants, general-purpose macros, the configuration
parameters of the system and library routines. It should be included in every application. For more
information refer to the Library Documentation File.
This file is mostly self-explaining. As already mentioned, the dt_comp library does not require any
configuration parameters. The following table presents the parameters used to initialise the PWM block .It may be
emphasized the dead time period set at 6μsec, a large value for the power devices used on the IRPT2056D.
{********************************************************************************************}
{ Library: PWM block }
{ file : PWM331.dsp }
{ Application Note: Usage of the ADMC331 Pulse Width Modulation Block }
.CONST PWM_freq = 10000; {Desired PWM switching frequency [Hz] }
.CONST PWM_deadtime = 6000; {Desired deadtime [nsec] }
.CONST PWM_minpulse = 1000; {Desired minimal pulse time [nsec] }
.CONST PWM_syncpulse = 1540; {Desired sync pulse time [nsec] }
.CONST Half_PWMTM = 1000*Cry_clock/PWM_freq/2;
{********************************************************************************************}
3.4 The program offset.dsp and its header offset.h
The current transducers introduce an offset that has to be evaluated, otherwise the sign of the currents
would be determined with large errors. For this reason, at the beginning of the program, for a certain
number of PWM cycles (in this particular case 128, but may be more or less depending on the system)
there are measured the A/D channels corresponding to the two phase currents, V1 and V2. The average of
all measurements constitutes the offset of that current. Of course, this procedure may be applied at every
channel, if the signal is zero at the beginning of the program.
The header file offset.h contains the macros that are used during this process. Generally, they call
subroutines presented in the file offset.dsp.
This file begins declaring the variables OffsetCounter, TempOffset1 and TempOffset2 used in these
routines.
a Compensating the dead time of voltage inverters with the ADMC331 AN331-50
© Analog Devices Inc., August 2000 Page 13 of 18
{***************************************************************************************
* Global Variables Defined in this Module *
***************************************************************************************}
.VAR/DM/RAM/SEG=USER_DM OffsetCounter[1];
.GLOBAL OffsetCounter;
.VAR/DM/RAM/CIRC/SEG=USER_DM TempOffset1[2];
.GLOBAL TempOffset1;
.VAR/DM/RAM/CIRC/SEG=USER_DM TempOffset2[2];
.GLOBAL TempOffset2;
The subroutine Offset_Init_ initialises the variables used to evaluate the offsets of the current transducers.
OffsetCounter is set to 128 because the offsets are considered the average of 128 measurements.
{*************************************************************************************
* Type: Routine *
* Call: Call Offset_Init_; *
* This subroutine initializes the variables initializes variables used to *
* evaluate the offsets of the current sensors *
* Inputs : None *
* Ouputs :None *
* Modified: AR *
***************************************************************************************}
Offset_Init_:
AR = Offset_Average;
dm(OffsetCounter) = AR;
AR = 0x0;
dm(TempOffset1) = AR;
dm(TempOffset1+1) = AR;
dm(TempOffset2) = AR;
dm(TempOffset2+1) = AR;
rts;
The subroutine EvaluateIs_offset_ computes the average of the measurements of a particular A/D channel.
{***************************************************************************************
* Type: Routine *
* Call: Call EvaluateIs_offset_; *
* This subroutine computes the average of the measurements of one A/D channel *
* Inputs : AR = the lecture of the A/D channel
* I1 = placed at the begining of the buffer which is averaged *
* M1 = 0, L1 = 0 *
* Ouputs :None *
* Modified: AY1, AY0, AR, SR, AX0 *
***************************************************************************************}
EvaluateIs_offset_:
AY1 = dm(I1, M1);
a Compensating the dead time of voltage inverters with the ADMC331 AN331-50
© Analog Devices Inc., August 2000 Page 14 of 18
AY0 = dm(I1, M1);
AR = 0x4000 - AR;
SR = ASHIFT AR BY -7 (HI);
AR = SR0 + AY0;
AX0 = AR, AR = SR1 + AY1 + C;
dm(I1, M1) = AR;
dm(I1, M1) = AX0;
RTS;
. In the file offset.h there is a macro Offset_Init that initialises the address generators at the current offsets
buffers and then calls the subroutine Offset_Init_ from offset.dsp.
{***************************************************************************************
* Type: Macro *
* Call: Offset_Init; *
* This macro initializes variables used to evaluate the offsets of the current sensors *
* Input: none *
* Output: none *
* Modified: AR *
***************************************************************************************}
.MACRO Offset_Init;
CALL Offset_Init_;
.ENDMACRO;
The macro EvaluateIs_offset reads one A/D channel and computes the average offset of that channel
calling the subroutine EvaluateIs_offset_.
{***************************************************************************************
* Type: Macro *
* Call: EvaluateIs_offset; *
* Routine to compute the offset of one phase *
* Input: %0=the targeted AD channel *
* %1=the offset structure dedicated to the phase *
* %1=most significant word *
* %1+1=less significant word *
* Output: Current Offset structure *
* Modified: *
***************************************************************************************}
.MACRO EvaluateIs_offset(%0, %1);
ADC_Read(%0);
I1 = ^%1;
M1 = 1;
L1 = %%1;
a Compensating the dead time of voltage inverters with the ADMC331 AN331-50
© Analog Devices Inc., August 2000 Page 15 of 18
CALL EvaluateIs_offset_;
.ENDMACRO;
The macro OffsetDetermination computes the offsets of the both A/D channels that measure the phase
currents.
{***************************************************************************************
* Type: Macro *
* Call: OffsetDetermination *
* Routine to compute the offsets introduced by the current sensors *
* Input: %0=ADC1 *
* %1=ADC2 *
* %2=Is1Offset *
* %3=Is2Offset *
* Output: Current Offsets structure *
* Modified: *
***************************************************************************************}
.MACRO OffsetDetermination(%0, %1, %2, %3);
AY0 = dm(OffsetCounter);
AR = AY0 - 1;
IF LT JUMP SaveOffsets;
dm(OffsetCounter) = AR;
EvaluateIs_offset(%0, TempOffset1);
EvaluateIs_offset(%1, TempOffset2);
RTI;
SaveOffsets:
AF = AR + 1;
IF NE JUMP ExitOffsetDet;
dm(OffsetCounter) = AR;
AR = dm(TempOffset1);
dm(%2) = AR;
AR = dm(TempOffset2);
dm(%3) = AR;
ExitOffsetDet:
.ENDMACRO;
The macro ReadCurrents reads the two phase currents, corrects them with the offset and finally computes
the third phase current. It may be noted that the output of the A/D converter is always a positive number. Because of
the presence of an inverting operational amplifier in the hardware, in order to obtain values between –1/2 and +1/2
(in fixed point the currents are scaled by 2⋅ Imax ) the outputs of the A/D converter have to be offset by 1/2
(0x4000).
{***************************************************************************************
* Type: Macro *
* Call: ReadCurrents; *
a Compensating the dead time of voltage inverters with the ADMC331 AN331-50
© Analog Devices Inc., August 2000 Page 16 of 18
* This macro reads ADC1(Is1), ADC2(Is2) and then evaluates Is1, Is2 and Is3 *
* Input: %0 = offset of the first phase current *
* %1 = offset of the second phase current *
* %2 = the buffer of the 3 phase currents *
* %3 = ADC1 *
* %4 = ADC2 *
* Output: none *
* Modified: AY0, AR, MY0, MR, SR ,AF *
***************************************************************************************}
.MACRO ReadCurrents(%0, %1, %2, %3, %4);
ADC_Read(%3); { read Is1/Imax }
AR = 0x4000 - AR;
AY0 = dm(%0);
AR = AR - AY0;
dm(%2) = AR; { Is1/2Imax }
ADC_Read(%4); { read Is2/Imax }
AR = 0x4000 - AR;
AY0 = dm(%1);
AR = AR - AY0;
dm(%2+1) = AR; { Is2/2Imax }
AR = -AR;
AY0 = dm(%2); { Is1/2Imax }
AR = AR - AY0;
dm(%2+2) = AR; { Is3/2Imax=-Is2/2Imax-Is1/2Imax}
.ENDMACRO;
3.5 Experimental results
First of all, experiments without the dead time compensation were made. Figure 4 represents the inverter
phase voltage compared to the reference voltage that is desired at the inverter terminal and the phase
current. It may be seen that the behavior presented in chapter 1 is verified in practice: When the phase
current is positive, the real inverter phase voltage is less than the commanded one by an amount
determined by the dead time and when the phase current is negative, the real inverter phase voltage is
greater than the commanded.
At last, Figure 5 displays the inverter phase voltage and the phase current obtained with the feed forward
dead time compensation. It may be observed that the voltage still presents some distortions caused by the
nature of feed forwarding: it is supposed that the current measured during the previous PWM cycle
maintains its sign into the next PWM cycle; when the current changes the sign, this moment cannot be
foreseen and the error is doubled. These voltage deformations cause also deformations in the current
behaviour, and they may be prevented only implementing current controllers in a more accurate control
strategy, like field-oriented control.
a Compensating the dead time of voltage inverters with the ADMC331 AN331-50
© Analog Devices Inc., August 2000 Page 17 of 18
Figure 4. Reference and real inverter phase voltages and the phase current
a Compensating the dead time of voltage inverters with the ADMC331 AN331-50
© Analog Devices Inc., August 2000 Page 18 of 18
Figure 5. Inverter phase voltage and phase current after the dead time compensation
4 References
[1] Pulse dead time compensator for PWM voltage inverters, David Leggate, Russel J. Kerkman,
Industrial Electronics, Control, and Instrumentation, 1995, Proceedings of the 1995 IEEE IECON 21st
International Conference on Volume: 1, Page(s): 474 -481 vol.1.
SN74HC4066
QUADRUPLE BILATERAL ANALOG SWITCH
SCLS325G – MARCH 1996 – REVISED JULY 2003
POST OFFICE BOX 655303 • DALLAS, TEXAS 75265 1
Wide Operating Voltage Range of 2 V to 6 V
Typical Switch Enable Time of 18 ns
Low Power Consumption, 20-μA Max ICC
Low Input Current of 1 μA Max
High Degree of Linearity
High On-Off Output-Voltage Ratio
Low Crosstalk Between Switches
Low On-State Impedance . . .
50-Ω TYP at VCC = 6 V
Individual Switch Controls
description/ordering information
The SN74HC4066 is a silicon-gate CMOS quadruple analog switch designed to handle both analog and digital
signals. Each switch permits signals with amplitudes of up to 6 V (peak) to be transmitted in either direction.
Each switch section has its own enable input control (C). A high-level voltage applied to C turns on the
associated switch section.
Applications include signal gating, chopping, modulation or demodulation (modem), and signal multiplexing for
analog-to-digital and digital-to-analog conversion systems.
ORDERING INFORMATION
TA PACKAGE† ORDERABLE
PART NUMBER
TOP-SIDE
MARKING
PDIP – N Tube of 25 SN74HC4066N SN74HC4066N
Tube of 50 SN74HC4066D
SOIC – D Reel of 2500 SN74HC4066DR HC4066
Reel of 250 SN74HC4066DT
–40°C to 85°C SOP – NS Reel of 2000 SN74HC4066NSR HC4066
SSOP – DB Reel of 2000 SN74HC4066DBR HC4066
Tube of 90 SN74HC4066PW
TSSOP – PW Reel of 2000 SN74HC4066PWR HC4066
Reel of 250 SN74HC4066PWT
† Package drawings, standard packing quantities, thermal data, symbolization, and PCB design
guidelines are available at www.ti.com/sc/package.
FUNCTION TABLE
(each switch)
INPUT
CONTROL
(C)
SWITCH
L OFF
H ON
PRODUCTION DATA information is current as of publication date. Copyright 2003, Texas Instruments Incorporated
Products conform to specifications per the terms of Texas Instruments
standard warranty. Production processing does not necessarily include
testing of all parameters.
Please be aware that an important notice concerning availability, standard warranty, and use in critical applications of
Texas Instruments semiconductor products and disclaimers thereto appears at the end of this data sheet.
1
2
3
4
5
6
7
14
13
12
11
10
9
8
1A
1B
2B
2A
2C
3C
GND
VCC
1C
4C
4A
4B
3B
3A
D, DB, N, NS, OR PW PACKAGE
(TOP VIEW)
SN74HC4066
QUADRUPLE BILATERAL ANALOG SWITCH
SCLS325G – MARCH 1996 – REVISED JULY 2003
2 POST OFFICE BOX 655303 • DALLAS, TEXAS 75265
logic diagram, each switch (positive logic)
A
VCC
VCC
B
One of Four Switches
C
absolute maximum ratings over operating free-air temperature range (unless otherwise noted)†
Supply voltage range, VCC (see Note 1) . . . . . . . . . . . . . . . . . . . . . . . . . . . . . . . . . . . . . . . . . . . . . . –0.5 V to 7 V
Control-input diode current, II (VI < 0 or VI > VCC) . . . . . . . . . . . . . . . . . . . . . . . . . . . . . . . . . . . . . . . . . . . ±20 mA
I/O port diode current, II (VI < 0 or VI/O > VCC) . . . . . . . . . . . . . . . . . . . . . . . . . . . . . . . . . . . . . . . . . . . . . . ±20 mA
On-state switch current (VI/O = 0 to VCC) . . . . . . . . . . . . . . . . . . . . . . . . . . . . . . . . . . . . . . . . . . . . . . . . . . ±25 mA
Continuous current through VCC or GND . . . . . . . . . . . . . . . . . . . . . . . . . . . . . . . . . . . . . . . . . . . . . . . . . . . ±50 mA
Package thermal impedance, θJA (see Note 2): D package . . . . . . . . . . . . . . . . . . . . . . . . . . . . . . . . . . . 86°C/W
DB package . . . . . . . . . . . . . . . . . . . . . . . . . . . . . . . . . 96°C/W
N package . . . . . . . . . . . . . . . . . . . . . . . . . . . . . . . . . . . 80°C/W
NS package . . . . . . . . . . . . . . . . . . . . . . . . . . . . . . . . . 76°C/W
PW package . . . . . . . . . . . . . . . . . . . . . . . . . . . . . . . . 113°C/W
Storage temperature range, Tstg . . . . . . . . . . . . . . . . . . . . . . . . . . . . . . . . . . . . . . . . . . . . . . . . . . . –65°C to 150°C
† Stresses beyond those listed under “absolute maximum ratings” may cause permanent damage to the device. These are stress ratings only, and
functional operation of the device at these or any other conditions beyond those indicated under “recommended operating conditions” is not
implied. Exposure to absolute-maximum-rated conditions for extended periods may affect device reliability.
NOTES: 1. All voltages are with respect to ground unless otherwise specified.
2. The package thermal impedance is calculated in accordance with JESD 51-7.
SN74HC4066
QUADRUPLE BILATERAL ANALOG SWITCH
SCLS325G – MARCH 1996 – REVISED JULY 2003
POST OFFICE BOX 655303 • DALLAS, TEXAS 75265 3
recommended operating conditions (see Note 3)
MIN NOM MAX UNIT
VCC Supply voltage 2† 5 6 V
VI/O I/O port voltage 0 VCC V
VCC = 2 V 1.5 VCC
VIH High-level input voltage, control inputs VCC = 4.5 V 3.15 VCC V
VCC = 6 V 4.2 VCC
VCC = 2 V 0 0.3
VIL Low-level input voltage, control inputs VCC = 4.5 V 0 0.9 V
VCC = 6 V 0 1.2
VCC = 2 V 1000
Δt/Δv Input transition rise/fall time VCC = 4.5 V 500 ns
VCC = 6 V 400
TA Operating free-air temperature –40 85 °C
† With supply voltages at or near 2 V, the analog switch on-state resistance becomes very nonlinear. It is recommended that only digital signals
be transmitted at these low supply voltages.
NOTE 3: All unused inputs of the device must be held at VCC or GND to ensure proper device operation. Refer to the TI application report,
Implications of Slow or Floating CMOS Inputs, literature number SCBA004.
electrical characteristics over recommended operating free-air temperature range (unless
otherwise noted)
PARAMETER TEST CONDITIONS V
TA = 25C
VCC MIN MAX UNIT
MIN TYP MAX
I A V 0t V
2 V 150
ron On-state switch resistance
IT = –1 mA, VI = 0 to VCC,
4.5 V 50 85 106 Ω VC = VIH (see Figure 1)
6 V 30
V V GND V V
2 V 320
ron(p) Peak on-state resistance
VI = VCC or GND, VC = VIH,
( ) 4.5 V 70 170 215 Ω IT = –1 mA
6 V 50
II Control input current VC = 0 or VCC 6 V ±0.1 ±100 ±1000 nA
Isoff Off-state switch leakage current
VI = VCC or 0, VO = VCC or 0,
VC = VIL (see Figure 2)
6 V ±0.1 ±5 μA
Ison On-state switch leakage current
VI = VCC or 0, VC = VIH
(see Figure 3)
6 V ±0.1 ±5 μA
ICC Supply current VI = 0 or VCC, IO = 0 6 V 2 20 μA
Ci Input capacitance
A or B
5 V
9
pF
C
3 10 10
Cf
Feed-through
capacitance
A to B VI = 0 0.5 pF
Co Output capacitance A or B 5 V 9 pF
SN74HC4066
QUADRUPLE BILATERAL ANALOG SWITCH
SCLS325G – MARCH 1996 – REVISED JULY 2003
4 POST OFFICE BOX 655303 • DALLAS, TEXAS 75265
switching characteristics over recommended operating free-air temperature range
PARAMETER
FROM TO TEST
VCC
TA = 25C
MIN MAX UNIT
(INPUT) (OUTPUT) CONDITIONS
MIN TYP MAX
t P ti C 50 F
2 V 10 60 75
tPLH,
Propagation
A or B B or A CL = pF
4.5 V 4 12 15 ns
tPHL
delay time
(see Figure 4)
6 V 3 10 13
t S it h RL = 1 kΩ,
2 V 70 180 225
tPZH,
tPZL
Switch
turn-on time
C A or B
CL = 50 pF 4.5 V 21 36 45 ns
L
(see Figure 5) 6 V 18 31 38
t S it h RL = 1 kΩ,
2 V 50 200 250
tPLZ,
Switch
C A or B
CL = 50 pF 4.5 V 25 40 50 ns
tPHZ
turn-off time
L
(see Figure 5) 6 V 22 34 43
Control
CL = 15 pF,
RL = 1 kΩ
2 V 15
fI
input
frequency
C A or B
kΩ,
VC = VCC or GND,
V V /2
4.5 V 30 MHz
VO = VCC/(see Figure 6) 6 V 30
Control
feed-through
C A or B
CL = 50 pF,
Rin = RL = 600 Ω,
VC = VCC or GND
4.5 V 15
mV
noise
GND,
fin = 1 MHz
(see Figure 7)
6 V 20
(rms)
operating characteristics, VCC = 4.5 V, TA = 25°C
PARAMETER TEST CONDITIONS TYP UNIT
Cpd Power dissipation capacitance per gate CL = 50 pF, f = 1 MHz 45 pF
Minimum through bandwidth, A to B or B to A† [20 log (VO/VI)] = –3 dB
CL = 50 pF,
VC = VCC
RL = 600 Ω,
(see Figure 8)
30 MHz
Crosstalk between any switches‡ CL = 10 pF,
fin = 1 MHz
RL = 50 Ω,
(see Figure 9)
45 dB
Feed through, switch off, A to B or B to A‡ CL = 50 pF,
fin = 1 MHz
RL = 600 Ω,
(see Figure 10)
42 dB
Amplitude distortion rate, A to B or B to A
CL = 50 pF,
fin = 1 kHz
RL = 10 kΩ,
(see Figure 11)
0.05%
† Adjust the input amplitude for output = 0 dBm at f = 1 MHz. Input signal must be a sine wave.
‡ Adjust the input amplitude for input = 0 dBm at f = 1 MHz. Input signal must be a sine wave.
SN74HC4066
QUADRUPLE BILATERAL ANALOG SWITCH
SCLS325G – MARCH 1996 – REVISED JULY 2003
POST OFFICE BOX 655303 • DALLAS, TEXAS 75265 5
PARAMETER MEASUREMENT INFORMATION
VCC
VI = VCC
VC = VIH
+ 1.0 mA –
VO
ron
VI–O
10–3
VI–O
VCC
GND
(ON)
V
Figure 1. On-State Resistance Test Circuit
VCC
VC = VIL
A B
VS = VA – VB
CONDITION 1: VA = 0, VB = VCC
CONDITION 2: VA = VCC, VB = 0
VCC
GND
A (OFF)
Figure 2. Off-State Switch Leakage-Current Test Circuit
SN74HC4066
QUADRUPLE BILATERAL ANALOG SWITCH
SCLS325G – MARCH 1996 – REVISED JULY 2003
6 POST OFFICE BOX 655303 • DALLAS, TEXAS 75265
PARAMETER MEASUREMENT INFORMATION
VCC
VC = VIH
A B
VCC Open
VA = VCC TO GND
VCC
GND
A (ON)
Figure 3. On-State Leakage-Current Test Circuit
VCC
VC = VIH
VI VO
50 pF
TEST CIRCUIT
tPLH tPHL
50% 50%
VCC
0 V
50% 50%
VOH
VOL
VI
A or B
VO
B or A
VOLTAGE WAVEFORMS
50 Ω
tr
90%
10%
tf
10%
90%
VCC
GND
(ON)
Figure 4. Propagation Delay Time, Signal Input to Signal Output
SN74HC4066
QUADRUPLE BILATERAL ANALOG SWITCH
SCLS325G – MARCH 1996 – REVISED JULY 2003
POST OFFICE BOX 655303 • DALLAS, TEXAS 75265 7
PARAMETER MEASUREMENT INFORMATION
CL
GND 50 pF
VCC
VI
VO
TEST CIRCUIT
tPLZ
50%
VOLTAGE WAVEFORMS
RL
1 kΩ
10%
S1
VC
50 Ω
S2
tPZH
tPHZ
50%
50%
50%
90%
tPZL
tPZH
tPLZ
tPHZ
GND
VCC
GND
VCC
TEST S1 S2
VCC
GND
VCC
GND
tPZL
50%
VCC
VO 50%
0 V
VOL
VOH
VC
(tPZL, tPZH)
(tPLZ, tPHZ)
VCC
VCC
VO
0 V
VOL
VOH
VC
VCC
0 V
VOL
VOH
VCC
0 V
VOL
VOH
Figure 5. Switching Time (tPZL, tPLZ, tPZH, tPHZ), Control to Signal Output
SN74HC4066
QUADRUPLE BILATERAL ANALOG SWITCH
SCLS325G – MARCH 1996 – REVISED JULY 2003
8 POST OFFICE BOX 655303 • DALLAS, TEXAS 75265
PARAMETER MEASUREMENT INFORMATION
VCC
GND
VO
RL
1 kΩ
CL
15 pF
VCC
VC
50 Ω
VI = VCC
VCC
VC
0 V
VCC/2
Figure 6. Control-Input Frequency
VCC
GND
VO
RL
600 Ω
CL
50 pF
VCC
VC
50 Ω
VI
VCC/2
Rin
600 Ω
VCC/2
tr tf
90%
10%
(f = 1 MHz)
tr = tf = 6 ns
90%
10%
VCC
VC
0 V
Figure 7. Control Feed-Through Noise
VO
VCC
50 Ω
fin
VCC/2
VC = VCC
0.1 μF VI VI
(VI = 0 dBm at f = 1 MHz)
VCC
GND
(ON)
RL
600 Ω
CL
50 pF
Figure 8. Minimum Through Bandwidth
SN74HC4066
QUADRUPLE BILATERAL ANALOG SWITCH
SCLS325G – MARCH 1996 – REVISED JULY 2003
POST OFFICE BOX 655303 • DALLAS, TEXAS 75265 9
PARAMETER MEASUREMENT INFORMATION
VO1
RL
600 Ω
CL
50 pF
VCC
50 Ω
fin
VCC/2
VC = VCC
0.1 μF
VI
VI
(VI = 0 dBm at f = 1 MHz)
VO2
VCC
Rin
600 Ω
VCC/2
VC = GND
Rin
600 Ω
VCC
GND
(ON)
VCC
GND
(OFF)
RL
600 Ω
CL
50 pF
Figure 9. Crosstalk Between Any Two Switches
VO
VCC
50 Ω
fin
VC = GND
0.1 μF VI VI
(VI = 0 dBm at f = 1 MHz)
VCC
GND
(OFF)
Rin
600 Ω
RL
600 Ω
CL
50 pF
VCC/2 VCC/2
Figure 10. Feed Through, Switch Off
VI
(VI = 0 dBm at f = 1 kHz)
VO
RL
10 kΩ
CL
50 pF
VCC
VCC/2
VC = VCC
10 μF
VI fin
VCC
GND
(ON)
Figure 11. Amplitude-Distortion Rate
PACKAGE OPTION ADDENDUM
www.ti.com 10-Jun-2014
Addendum-Page 1
PACKAGING INFORMATION
Orderable Device Status
(1)
Package Type Package
Drawing
Pins Package
Qty
Eco Plan
(2)
Lead/Ball Finish
(6)
MSL Peak Temp
(3)
Op Temp (°C) Device Marking
(4/5)
Samples
SN74HC4066D ACTIVE SOIC D 14 50 Green (RoHS
& no Sb/Br)
CU NIPDAU Level-1-260C-UNLIM -40 to 85 HC4066
SN74HC4066DBLE OBSOLETE SSOP DB 14 TBD Call TI Call TI -40 to 85
SN74HC4066DBR ACTIVE SSOP DB 14 2000 Green (RoHS
& no Sb/Br)
CU NIPDAU Level-1-260C-UNLIM -40 to 85 HC4066
SN74HC4066DBRE4 ACTIVE SSOP DB 14 2000 Green (RoHS
& no Sb/Br)
CU NIPDAU Level-1-260C-UNLIM -40 to 85 HC4066
SN74HC4066DG4 ACTIVE SOIC D 14 50 Green (RoHS
& no Sb/Br)
CU NIPDAU Level-1-260C-UNLIM -40 to 85 HC4066
SN74HC4066DR ACTIVE SOIC D 14 2500 Green (RoHS
& no Sb/Br)
CU NIPDAU Level-1-260C-UNLIM -40 to 85 HC4066
SN74HC4066DRE4 ACTIVE SOIC D 14 2500 Green (RoHS
& no Sb/Br)
CU NIPDAU Level-1-260C-UNLIM -40 to 85 HC4066
SN74HC4066DRG4 ACTIVE SOIC D 14 2500 Green (RoHS
& no Sb/Br)
CU NIPDAU Level-1-260C-UNLIM -40 to 85 HC4066
SN74HC4066DT ACTIVE SOIC D 14 250 Green (RoHS
& no Sb/Br)
CU NIPDAU Level-1-260C-UNLIM -40 to 85 HC4066
SN74HC4066N ACTIVE PDIP N 14 25 Pb-Free
(RoHS)
CU NIPDAU N / A for Pkg Type -40 to 85 SN74HC4066N
SN74HC4066NE4 ACTIVE PDIP N 14 25 Pb-Free
(RoHS)
CU NIPDAU N / A for Pkg Type -40 to 85 SN74HC4066N
SN74HC4066NSR ACTIVE SO NS 14 2000 Green (RoHS
& no Sb/Br)
CU NIPDAU Level-1-260C-UNLIM -40 to 85 HC4066
SN74HC4066PW ACTIVE TSSOP PW 14 90 Green (RoHS
& no Sb/Br)
CU NIPDAU Level-1-260C-UNLIM -40 to 85 HC4066
SN74HC4066PWG4 ACTIVE TSSOP PW 14 90 Green (RoHS
& no Sb/Br)
CU NIPDAU Level-1-260C-UNLIM -40 to 85 HC4066
SN74HC4066PWLE OBSOLETE TSSOP PW 14 TBD Call TI Call TI -40 to 85
SN74HC4066PWR ACTIVE TSSOP PW 14 2000 Green (RoHS
& no Sb/Br)
CU NIPDAU Level-1-260C-UNLIM -40 to 85 HC4066
SN74HC4066PWRG4 ACTIVE TSSOP PW 14 2000 Green (RoHS
& no Sb/Br)
CU NIPDAU Level-1-260C-UNLIM -40 to 85 HC4066
SN74HC4066PWT ACTIVE TSSOP PW 14 250 Green (RoHS
& no Sb/Br)
CU NIPDAU Level-1-260C-UNLIM -40 to 85 HC4066
PACKAGE OPTION ADDENDUM
www.ti.com 10-Jun-2014
Addendum-Page 2
(1) The marketing status values are defined as follows:
ACTIVE: Product device recommended for new designs.
LIFEBUY: TI has announced that the device will be discontinued, and a lifetime-buy period is in effect.
NRND: Not recommended for new designs. Device is in production to support existing customers, but TI does not recommend using this part in a new design.
PREVIEW: Device has been announced but is not in production. Samples may or may not be available.
OBSOLETE: TI has discontinued the production of the device.
(2) Eco Plan - The planned eco-friendly classification: Pb-Free (RoHS), Pb-Free (RoHS Exempt), or Green (RoHS & no Sb/Br) - please check http://www.ti.com/productcontent for the latest availability
information and additional product content details.
TBD: The Pb-Free/Green conversion plan has not been defined.
Pb-Free (RoHS): TI's terms "Lead-Free" or "Pb-Free" mean semiconductor products that are compatible with the current RoHS requirements for all 6 substances, including the requirement that
lead not exceed 0.1% by weight in homogeneous materials. Where designed to be soldered at high temperatures, TI Pb-Free products are suitable for use in specified lead-free processes.
Pb-Free (RoHS Exempt): This component has a RoHS exemption for either 1) lead-based flip-chip solder bumps used between the die and package, or 2) lead-based die adhesive used between
the die and leadframe. The component is otherwise considered Pb-Free (RoHS compatible) as defined above.
Green (RoHS & no Sb/Br): TI defines "Green" to mean Pb-Free (RoHS compatible), and free of Bromine (Br) and Antimony (Sb) based flame retardants (Br or Sb do not exceed 0.1% by weight
in homogeneous material)
(3) MSL, Peak Temp. - The Moisture Sensitivity Level rating according to the JEDEC industry standard classifications, and peak solder temperature.
(4) There may be additional marking, which relates to the logo, the lot trace code information, or the environmental category on the device.
(5) Multiple Device Markings will be inside parentheses. Only one Device Marking contained in parentheses and separated by a "~" will appear on a device. If a line is indented then it is a continuation
of the previous line and the two combined represent the entire Device Marking for that device.
(6) Lead/Ball Finish - Orderable Devices may have multiple material finish options. Finish options are separated by a vertical ruled line. Lead/Ball Finish values may wrap to two lines if the finish
value exceeds the maximum column width.
Important Information and Disclaimer:The information provided on this page represents TI's knowledge and belief as of the date that it is provided. TI bases its knowledge and belief on information
provided by third parties, and makes no representation or warranty as to the accuracy of such information. Efforts are underway to better integrate information from third parties. TI has taken and
continues to take reasonable steps to provide representative and accurate information but may not have conducted destructive testing or chemical analysis on incoming materials and chemicals.
TI and TI suppliers consider certain information to be proprietary, and thus CAS numbers and other limited information may not be available for release.
In no event shall TI's liability arising out of such information exceed the total purchase price of the TI part(s) at issue in this document sold by TI to Customer on an annual basis.
TAPE AND REEL INFORMATION
*All dimensions are nominal
Device Package
Type
Package
Drawing
Pins SPQ Reel
Diameter
(mm)
Reel
Width
W1 (mm)
A0
(mm)
B0
(mm)
K0
(mm)
P1
(mm)
W
(mm)
Pin1
Quadrant
SN74HC4066DBR SSOP DB 14 2000 330.0 16.4 8.2 6.6 2.5 12.0 16.0 Q1
SN74HC4066DR SOIC D 14 2500 330.0 16.4 6.5 9.0 2.1 8.0 16.0 Q1
SN74HC4066DT SOIC D 14 250 330.0 16.4 6.5 9.0 2.1 8.0 16.0 Q1
SN74HC4066NSR SO NS 14 2000 330.0 16.4 8.2 10.5 2.5 12.0 16.0 Q1
SN74HC4066PWR TSSOP PW 14 2000 330.0 12.4 6.9 5.6 1.6 8.0 12.0 Q1
SN74HC4066PWT TSSOP PW 14 250 330.0 12.4 6.9 5.6 1.6 8.0 12.0 Q1
PACKAGE MATERIALS INFORMATION
www.ti.com 14-Jul-2012
Pack Materials-Page 1
*All dimensions are nominal
Device Package Type Package Drawing Pins SPQ Length (mm) Width (mm) Height (mm)
SN74HC4066DBR SSOP DB 14 2000 367.0 367.0 38.0
SN74HC4066DR SOIC D 14 2500 367.0 367.0 38.0
SN74HC4066DT SOIC D 14 250 367.0 367.0 38.0
SN74HC4066NSR SO NS 14 2000 367.0 367.0 38.0
SN74HC4066PWR TSSOP PW 14 2000 367.0 367.0 35.0
SN74HC4066PWT TSSOP PW 14 250 367.0 367.0 35.0
PACKAGE MATERIALS INFORMATION
www.ti.com 14-Jul-2012
Pack Materials-Page 2
MECHANICAL DATA
MSSO002E – JANUARY 1995 – REVISED DECEMBER 2001
POST OFFICE BOX 655303 • DALLAS, TEXAS 75265
DB (R-PDSO-G**) PLASTIC SMALL-OUTLINE
4040065 /E 12/01
28 PINS SHOWN
Gage Plane
8,20
7,40
0,55
0,95
0,25
38
12,90
12,30
28
10,50
24
8,50
Seating Plane
7,90 9,90
30
10,50
9,90
0,38
5,60
5,00
15
0,22
14
A
28
1
16 20
6,50 6,50
14
0,05 MIN
5,90 5,90
DIM
A MAX
A MIN
PINS **
2,00 MAX
6,90
7,50
0,65 0,15 M
0°–8°
0,10
0,09
0,25
NOTES: A. All linear dimensions are in millimeters.
B. This drawing is subject to change without notice.
C. Body dimensions do not include mold flash or protrusion not to exceed 0,15.
D. Falls within JEDEC MO-150
IMPORTANT NOTICE
Texas Instruments Incorporated and its subsidiaries (TI) reserve the right to make corrections, enhancements, improvements and other
changes to its semiconductor products and services per JESD46, latest issue, and to discontinue any product or service per JESD48, latest
issue. Buyers should obtain the latest relevant information before placing orders and should verify that such information is current and
complete. All semiconductor products (also referred to herein as “components”) are sold subject to TI’s terms and conditions of sale
supplied at the time of order acknowledgment.
TI warrants performance of its components to the specifications applicable at the time of sale, in accordance with the warranty in TI’s terms
and conditions of sale of semiconductor products. Testing and other quality control techniques are used to the extent TI deems necessary
to support this warranty. Except where mandated by applicable law, testing of all parameters of each component is not necessarily
performed.
TI assumes no liability for applications assistance or the design of Buyers’ products. Buyers are responsible for their products and
applications using TI components. To minimize the risks associated with Buyers’ products and applications, Buyers should provide
adequate design and operating safeguards.
TI does not warrant or represent that any license, either express or implied, is granted under any patent right, copyright, mask work right, or
other intellectual property right relating to any combination, machine, or process in which TI components or services are used. Information
published by TI regarding third-party products or services does not constitute a license to use such products or services or a warranty or
endorsement thereof. Use of such information may require a license from a third party under the patents or other intellectual property of the
third party, or a license from TI under the patents or other intellectual property of TI.
Reproduction of significant portions of TI information in TI data books or data sheets is permissible only if reproduction is without alteration
and is accompanied by all associated warranties, conditions, limitations, and notices. TI is not responsible or liable for such altered
documentation. Information of third parties may be subject to additional restrictions.
Resale of TI components or services with statements different from or beyond the parameters stated by TI for that component or service
voids all express and any implied warranties for the associated TI component or service and is an unfair and deceptive business practice.
TI is not responsible or liable for any such statements.
Buyer acknowledges and agrees that it is solely responsible for compliance with all legal, regulatory and safety-related requirements
concerning its products, and any use of TI components in its applications, notwithstanding any applications-related information or support
that may be provided by TI. Buyer represents and agrees that it has all the necessary expertise to create and implement safeguards which
anticipate dangerous consequences of failures, monitor failures and their consequences, lessen the likelihood of failures that might cause
harm and take appropriate remedial actions. Buyer will fully indemnify TI and its representatives against any damages arising out of the use
of any TI components in safety-critical applications.
In some cases, TI components may be promoted specifically to facilitate safety-related applications. With such components, TI’s goal is to
help enable customers to design and create their own end-product solutions that meet applicable functional safety standards and
requirements. Nonetheless, such components are subject to these terms.
No TI components are authorized for use in FDA Class III (or similar life-critical medical equipment) unless authorized officers of the parties
have executed a special agreement specifically governing such use.
Only those TI components which TI has specifically designated as military grade or “enhanced plastic” are designed and intended for use in
military/aerospace applications or environments. Buyer acknowledges and agrees that any military or aerospace use of TI components
which have not been so designated is solely at the Buyer's risk, and that Buyer is solely responsible for compliance with all legal and
regulatory requirements in connection with such use.
TI has specifically designated certain components as meeting ISO/TS16949 requirements, mainly for automotive use. In any case of use of
non-designated products, TI will not be responsible for any failure to meet ISO/TS16949.
Products Applications
Audio www.ti.com/audio Automotive and Transportation www.ti.com/automotive
Amplifiers amplifier.ti.com Communications and Telecom www.ti.com/communications
Data Converters dataconverter.ti.com Computers and Peripherals www.ti.com/computers
DLP® Products www.dlp.com Consumer Electronics www.ti.com/consumer-apps
DSP dsp.ti.com Energy and Lighting www.ti.com/energy
Clocks and Timers www.ti.com/clocks Industrial www.ti.com/industrial
Interface interface.ti.com Medical www.ti.com/medical
Logic logic.ti.com Security www.ti.com/security
Power Mgmt power.ti.com Space, Avionics and Defense www.ti.com/space-avionics-defense
Microcontrollers microcontroller.ti.com Video and Imaging www.ti.com/video
RFID www.ti-rfid.com
OMAP Applications Processors www.ti.com/omap TI E2E Community e2e.ti.com
Wireless Connectivity www.ti.com/wirelessconnectivity
Mailing Address: Texas Instruments, Post Office Box 655303, Dallas, Texas 75265
Copyright © 2014, Texas Instruments Incorporated
a Using a Tracebuffer with the ADMCF32X ANF32X-34
© Analog Devices Inc., March 2000 Page 1 of 11
a
Using a Tracebuffer with the
ADMCF32X
ANF32X-34
a Using a Tracebuffer with the ADMCF32X ANF32X-34
© Analog Devices Inc., March 2000 Page 2 of 11
Table of Contents
SUMMARY...................................................................................................................... 3
1 THE TRACEBUFFER STRUCTURE........................................................................ 3
1.1 The Tracebuffer Data-Array.........................................................................................................................4
2 IMPLEMENTATION OF THE TRACEBUFFER LIBRARY ROUTINES ................... 5
2.1 Usage of the tracebuffer routines ..................................................................................................................5
2.2 Usage of the DSP registers .............................................................................................................................5
2.3 Access to the library: the header file.............................................................................................................6
2.4 The program macro........................................................................................................................................7
3 SOFTWARE EXAMPLE: TRACEBUFFER.............................................................. 8
3.1 Usage of the Tracebuffer routine an example ..............................................................................................8
3.2 The main program: main.dsp........................................................................................................................8
4 EXPERIMENTAL RESULTS.................................................................................. 10
a Using a Tracebuffer with the ADMCF32X ANF32X-34
© Analog Devices Inc., March 2000 Page 3 of 11
Summary
In many cases the plotting and processing data externally are needed to verify and debug code and
structure in a DSP. This application note describe the use of a tracebuffer structure where values treated in
the DSP can be saved in a data-array and used for internal of external modification interfaced though the
Motion Control Debugger system.
1 The Tracebuffer Structure
A data-array structure is defined to enable saving arrays of values in data-memory (DM). This array of
memory locations can be addressed by the use of the pointer-system on the 2171 core. With this structure
defined, further treating or evaluation of the internal data-calculations can be analyzed and checked for
errors.
Using the Motion Control Debugger the values can be either be plotted directly or dumped for analyzing
the data-array in other external programs
In the chosen structure any number of pointer arrays in DM can be enabled and individually initialized for
locations in DM. The structure will furthermore allow the user to under-sample the writing to the buffer.
Initialize
the Tracebuffer
Though macro
Is the Sample Ratio = Sample number?
Is Flag enabled ?
Is there still space in the Buffer Full ?
YES
No
YES
No
Update Buffer and increment
pointer and counter
End Macro;
Macro Call
YES
No
Figure 1 - Flowchart for the Buffer writing
The flow chart illustrate the structure of the trace buffer writing. Initialization is done in the startup
sequence. After this, the Flag is checked - is the flag set then the corresponding tracebuffer is enabled.
Secondly the buffer is checked for available spaces. If the DM locations defined for memory write aren't
full it is safe to go on. If the buffer is full return. Finally the sample-ratio is checked. If a sample-ratio is
a Using a Tracebuffer with the ADMCF32X ANF32X-34
© Analog Devices Inc., March 2000 Page 4 of 11
declared different from zero then check if the sample-number is equal to the sample-ratio. If it is write the
chosen variable to the data-array. If not, return to the subroutine.
The structure of the buffer is circular and to optimize the flexibility the format is provided as a complete
macro setting with locked data-array format.
1.1 The Tracebuffer Data-Array
To enable the tracebuffer array in DM it is necessary to define a given circular buffer with associated
pointer. The circular buffer is structured as:
First location : Statement of flag - ON/OFF
Second location : Pointer to next free address
Third location : Sample ratio (specified by the user)
Fourth location : Sample number (used during the re-sampling of values)
Fifth location : Counter for the buffer.
Sixth to XXX locations : Placement for the values
Every time the macro is called, Ex. in the PWMSYNC_ISR, a new value is added to the buffer if there are
available space left and the sample number is equal to the under-sample ratio.
DM(Address) Flag (ON/OFF)
DM(Address+1) Pointer to next free
address
.. Sample ratio
.. Sample number
.. Counter for Buffer
.. First Data-placement
.. Value(1)
.. Value(2)
..
..
..
..
..
..
.. Value (Buffer size -2)
.. Value (Buffer size -1)
.. Value (Buffer size)
Figure 2 - Tracebuffer - locations in DM
Figure 2 illustrates how the values are placed in the allocated DM locations. Here values are stored at
specific addresses in order to analyze these off-line.
First value
Placed in the
buffer
N = numbers
in
tracebuffer
Buffer full
a Using a Tracebuffer with the ADMCF32X ANF32X-34
© Analog Devices Inc., March 2000 Page 5 of 11
2 Implementation of the Tracebuffer Library Routines
2.1 Usage of the tracebuffer routines
The routines are developed as an easy-to-use library, which has to be linked to the user’s application. The
library consists of two files. The file “T_buffer.dsp” contains the assembly code for the subroutines. This
package has to be compiled and can then be linked to an application. The user has to include the header
file “T_buffer.h”, which provides the function-like macros for this routine. The following table
summarizes the set of macros that are defined in this library.
Operation Usage Input Output
Initialization Buffer_Init("name", sample ratio);
Name &
Sample
ratio
None
Activate Buffer_ON("name"); Name None
Deactivate Buffer_OFF("name"); Name None
Record Buffer_Record("name", value);
Name &
Value
None
Table 1: Implemented routines
The four-macro settings allow the user to setup any given DM-locations for trace-buffer availability.
Specifying the selected buffer and record value enables the flexibility of writing any number to a known
position in memory.
2.2 Usage of the DSP registers
Table 2 gives an overview of the DSP core registers that are modified by the four macros mentioned
above. Obviously, also the "input" values are modified.
Usage Modified registers
Buffer_Init("name", sample ratio); ax0
Buffer_OFF("name"); ax0
Buffer_ON("name"); ax0
Buffer_Record("name", value);
ax0, ax1, ay0, ar,
I5, M5
Table 2: Usage of DSP core registers for the subroutines
a Using a Tracebuffer with the ADMCF32X ANF32X-34
© Analog Devices Inc., March 2000 Page 6 of 11
2.3 Access to the library: the header file
Including the header file "t_buffer.h" into the application code may access the library.
The header file is intended to provide function-like macros to the Trace buffer routines. It defines the calls
shown in Table 1. The file is mostly self-explaining but some comments have to be added. The sample ratio is
here defined as how often is a new value can be written to the buffer.
First macro is the Buffer_Init macro. This macro initializes the five first location of the circular buffer in
respect to "name of the buffer" and the sample-ratio. Furthermore the sample-number and the internal
counter is cleared.
The second and third macro Buffer_ON and Buffer_OFF just enables or disables writing to the buffers. In
this case the first location in the buffer ( the flag ) are set/or cleared.
{********************************************************************************
* *
* Type: Macro *
* *
* Call: Buffer_Init("Buffer", sampleratio) *
* Description : Initialize the tracebuffer *
* *
* Undersample ratio 0 = every time *
* 1 = every 1.time *
* 2 = every 2.time ..... *
* *
* Ouputs : none *
* *
* Modified: ax0 *
* *
********************************************************************************}
.MACRO Buffer_Init(%0,%1);
ax0 = %1; { Sample ratio }
dm(%0+2)= ax0;
ax0 =^%0+5; { Store start value }
dm(%0+1)= ax0; { first location for data }
ax0 = 0x0000;
dm(%0) = ax0; { Clear Flag - Non-Active }
dm(%0+3)= ax0; { Clear sample number }
dm(%0+4)= ax0; { Clear counter for this buffer }
.ENDMACRO;
{********************************************************************************
* *
* Type: Macro *
* *
* Call: Buffer_ON("buffer") *
* *
* Description : Enable tracebuffer "Buffer" *
* Ouputs : none *
* *
* Modified : ax0 *
* *
********************************************************************************}
.MACRO Buffer_ON(%0);
ax0 = 1;
dm(%0) = ax0;
.ENDMACRO;
a Using a Tracebuffer with the ADMCF32X ANF32X-34
© Analog Devices Inc., March 2000 Page 7 of 11
{********************************************************************************
* *
* Type: Macro *
* *
* Call: Buffer_OFF("buffer") *
* *
* Description : Disable tracebuffer "Buffer" *
* Ouputs : none *
* *
* Modified : ax0 *
* *
********************************************************************************}
.MACRO Buffer_OFF(%0);
ax0 = 0;
dm(%0) = ax0;
.ENDMACRO;
2.4 The program macro
The following code contained in the file “t_buffer.h” defines the macrocode used for the Tracebuffer. In
many cases this piece of code is placed in the "t_buffer.dsp"-file but here the flexibility advances by
placing the program-code directly in the macro. It should be mentioned that this way of using the
tracebuffer enables flexibility but takes up more memory.
The following code implements the tracebuffer routines. Refer to the flowchart in section 1 for the structure
of the buffers. Input to the tracebuffer are any numbers computed in the DSP.
Underneath is the code for the Buffer_Record.. It just need to be said that since the buffer is structured as a
circular buffer the data-placement for each of the "buffer-handle" values are placed from buffer-location 1 to
5 (here %0….%0+4)
{********************************************************************************
* *
* Type: Macro *
* *
* all: Buffer_Record(buffer,data) *
* *
* Description : Place data in buffer memory *
* Ouputs : none *
* *
* Modified: M5, I5, ar, ax1, ax0, ay0 *
* *
********************************************************************************}
.MACRO Buffer_Record(%0,%1);
.Local Continue1,Continue2,Continue3,End; { Local routines in Macro }
M5 = 1; { modify factor = 1 }
ax1 = %1;
I5 = ^%0; { load start value for pointer }
ar = dm(%0); { temporary storage }
ar= tstbit 0 of ar;
if NE jump Continue1;
Jump end;
Continue1:
ax0 = %%0;
ay0 = dm(%0+4);
ar = ax0 - ay0;
if gt jump Continue2;
ax0 = 0x0000;
dm(%0) = ax0;
Jump end;
a Using a Tracebuffer with the ADMCF32X ANF32X-34
© Analog Devices Inc., March 2000 Page 8 of 11
Continue2: { is sample_num equal to ratio? }
ax0 = dm(%0+3);
ay0 = dm(%0+2);
ar = ax0 - ay0;
if eq jump Continue3;
ar = ax0 + 1;
dm(%0+3) = ar;
Jump end;
Continue3: { write into buffer }
I5 = dm(%0+1); { load backup value for pointer }
dm(I5,M5) = ax1; { Value updated to Buffer }
ax0 = dm(%0+4); { increment count }
ar = ax0 + 1;
dm(%0+4) = ar;
ax0 = 0x0000; { clear sample_num }
dm(%0+3) = ax0;
dm(%0+1) = I5;
end:
.ENDMACRO;
3 Software Example: Tracebuffer
3.1 Usage of the Tracebuffer routine an example
This example demonstrates how two values are written to Buffer1 and Buffer2. In this case the memorylocations
used as buffers are set to 2*105-locations (100 location of calculated data). The values written
to these two buffer-arrays are values computed for three 120-degree phase shifted reference voltages.
3.2 The main program: main.dsp
The file “main.dsp” contains the initialisation and PWM Sync and Trip interrupt service routines. To
activate, build the executable file using the attached build.bat either within your DOS prompt or clicking
on it from Windows Explorer. This will create the object files and the main.exe example file. This file
may be run on the Motion Control Debugger. The program can be booted from Flash but in this
tracebuffer case it is not effectuated since the DM can not be read without the Motion Control Debugger.
Every module besides from the Main_program module is by default placed in either one of the three
USERFLASH memory banks.
In the following, a brief description of the code is given.
Start of code – declaring start location in program memory or FLASH memory. Comments are placed
depending on whether the program should run in PMRAM or Flash memory.
{**************************************************************************************
* Application: Starting from FLASH (out-comment the one not used)
**************************************************************************************}
!.MODULE/RAM/SEG=USERFLASH1/ABS=0x2200 Main_Program;
{**************************************************************************************
* Application: Starting from RAM (out-comment the one not used)
**************************************************************************************}
.MODULE/RAM/SEG=USER_PM1/ABS=0x30 Main_Program;
a Using a Tracebuffer with the ADMCF32X ANF32X-34
© Analog Devices Inc., March 2000 Page 9 of 11
Next, the general systems constants and PWM configuration constants (main.h – see the next section) are
included. Also included are the PWM library and the T_BUFFER library definitions
{********************************************************************************
* Include General System Parameters and Libraries *
********************************************************************************}
#include ;
#include ;
#include ;
#include ;
{********************************************************************************
* Local Variables Defined in this Module *
********************************************************************************}
.VAR/DM/RAM/SEG=USER_DM AD_IN; { Volts/Hertz Command (0-1) }
.VAR/DM/RAM/SEG=USER_DM Theta; { Current angle }
.VAR/DM/RAM/SEG=USER_DM VrefA; { Voltage demands }
.VAR/DM/RAM/SEG=USER_DM VrefB;
.VAR/DM/RAM/SEG=USER_DM VrefC;
.VAR/DM/RAM/CIRC/SEG=USER_DM Buffer1[105]; { Tracebuffer }
.VAR/DM/RAM/CIRC/SEG=USER_DM Buffer2[105]; { Tracebuffer }
ar = 0x7FFF; dm(AD_IN) = ar;
ar = 0x0000; dm(Theta) = ar; dm(VrefA) = ar; dm(VrefB) = ar; dm(VrefC) = ar;
Some Variables are defined hereafter. These are used to calculate the three reference voltages. For further
information see ANF32X-3. The two circular buffers are defined - here the size is 105 locations (5 locations
are used for handling the buffer) this number is arbitrary - just depending on the memory locations occupied
by these buffers.
The first thing that is done in the initialisation block (Startup) is checking a selected PIO line for level. If the
PIO-pin is high jump to an ERASE BOOT FROM FLASH BIT routine in ROM and return. If not, just go
ahead with normal operation. This small macro is done to enable re-coding of the FLASH memory. For
further information (See Reference Manual). In this example the PIO-pin 6 is chosen as erase pin. The
initialisation of the PWM block is executed. Note how the interrupt vectors for the PWMSync and PWMTrip
service routines are passed as arguments. Then the interrupt IRQ2 is enabled by setting the corresponding
bit in the IMASK register. Two Tracebuffers are initialised with 1x under-sampling Then the Tracebuffers
are activated by setting the flag (Buffer_ON(Buffer1) & Buffer_ON(Buffer2)). After that, the program
enters a loop, which just waits for interrupts.
{********************************************************************************
* Start of program code *
********************************************************************************}
Startup:
FLASH_erase_PIO(6); { Select PIO6 as clearing PIO }
{ Remember that sport1 is muxed with the PIO-lines }
{ If the bit is high Clear Memory and Boot from }
{ Flash bit }
PWM_Init(PWMSYNC_ISR, PWMTRIP_ISR);
IFC = 0x80; { Clear any pending IRQ2 inter. }
ay0 = 0x200; { unmask irq2 interrupts. }
ar = IMASK;
ar = ar or ay0;
IMASK = ar; { IRQ2 ints fully enabled here }
Buffer_Init(Buffer1, 1); { 1x undersampling }
Buffer_Init(Buffer2, 1); { 1X undersampling }
Buffer_ON(Buffer1); { Activate the Buffer }
Buffer_ON(Buffer2); { Activate the Buffer }
Main: { Wait for interrupt to occur }
jump Main;
rts;
a Using a Tracebuffer with the ADMCF32X ANF32X-34
© Analog Devices Inc., March 2000 Page 10 of 11
In the PWMSYNC_ISR the DAGS are first set up for trigonometric functionality. Three reference voltages
VrefA,B and C are calculated on base of the trigonometric functions in the Trigonometric-library ( See
ANF32X-10 ). The PWM block is update with these control signals and finally the two Tracebuffers Buffer1
and Buffer2 are updated. Here the variables VrefA and VrefB are stored in the two data-arrays.
PWMSYNC_ISR:
Set_DAG_registers_for_trigonometric;
my0 = DM(AD_IN);
mr = 0; { Clear mr }
mr1 = dm(Theta); { Preload Theta }
mx0 = Delta;
mr = mr + mx0*my0 (SS); { Compute new angle & store }
dm(Theta) = mr1;
Sin(mr1); { Result in ar register }
mr = ar*my0 (SS); { Multiply by Scale for VrefA }
dm(VrefA) = mr1;
ax1 = dm(Theta); { Compute angle of phase B }
ay1 = TwoPioverThree;
ar = ax1 - ay1;
Sin(ar); { Result in ar register }
mr = ar*my0 (SS); { Multiply by Scale for VrefB }
dm(VrefB) = mr1;
ax1 = dm(Theta); { Compute angle of phase C }
ay1 = TwoPioverThree;
ar = ax1 + ay1;
Sin(ar); { Result in ar register }
mr = ar*my0 (SS); { Multiply by Scale for VrefC }
dm(VrefC) = mr1;
ax0 = DM(VrefA); ax1 = DM(VrefB); ay0 = DM(VrefC); ay1= DM(Theta);
PWM_update_demanded_Voltage(ax0,ax1,ay0);
{*******************************************************************************
* Update tracebuffers *
*******************************************************************************}
ax0 = DM(VrefA); Buffer_Record(Buffer1,ax0);
ax0 = DM(VrefB); Buffer_Record(Buffer2,ax0);
RTI;
It has to be mentioned that the Buffer_Record macro uses some DSP registers (see T_buffer.h) for that
reason the proposed way of writing to the buffer is as defined above.
4 Experimental results
The experimental results illustrated beneath are two plots of VrefA and VrefB. These values are written
into Buffer1 and Buffer2 and then plotted though the Motion Control Debugger. As can be seen on Figure
3 the two waveforms are plotted as a function of the given number in Buffer1 and 2.
From the figures the scaling can also be seen - here the numbers are represented in decimal. Selecting
another scaling of these reference-voltages will re-scale these plots.
a Using a Tracebuffer with the ADMCF32X ANF32X-34
© Analog Devices Inc., March 2000 Page 11 of 11
Figure 3 - Plot from the Motion Control Debugger using the Internal Plot Function.
www.analog.com
Developing VisualAudio Modules
Copyright Information
© 2006 Analog Devices, Inc., ALL RIGHTS RESERVED. This document may not be reproduced in any form without prior, express written consent from Analog Devices, Inc.
Printed in the USA.
Disclaimer
Analog Devices, Inc. reserves the right to change this product without prior notice. Information furnished by Analog Devices is believed to be accurate and reliable. However, no responsibility is assumed by Analog Devices for its use; nor for any infringement of patents or other rights of third parties which may result from its use. No license is granted by implication or otherwise under the patent rights of Analog Devices, Inc.
Trademark and Service Mark Notice
The Analog Devices logo, VisualDSP++, VisualAudio, SHARC, Blackfin, and EZ-KIT Lite are registered trademarks of Analog Devices, Inc.
All other brand and product names are trademarks or service marks of their respective owners.
2 of 51
Contents
Contents..............................................................................................................................................................................................................3
Preface.................................................................................................................................................................................................................4
Purpose of This Manual................................................................................................................................................................................4
Custom Audio Modules....................................................................................................................................................................................5
Overview.........................................................................................................................................................................................................5
Numerics on the Blackfin and SHARC.......................................................................................................................................................9
Example 1A – Mono Parametric Scaling....................................................................................................................................................9
Example 1B – Render Function in ASM.................................................................................................................................................19
Scratch Buffers............................................................................................................................................................................................22
Auxiliary Memory for Module Instances................................................................................................................................................22
Pointer Aliasing Rules................................................................................................................................................................................25
Meta-Variables and Expressions...............................................................................................................................................................26
Modifying Module Parameters.................................................................................................................................................................27
Expression Language Details.....................................................................................................................................................................28
Modules With Data of Varying Size.........................................................................................................................................................33
Modules With a Variable Number of Pins...............................................................................................................................................34
Frequency Domain Processing.................................................................................................................................................................36
Other Features of the XML File................................................................................................................................................................36
Custom Bypass Functions..........................................................................................................................................................................38
SHARC SIMD Considerations..................................................................................................................................................................38
Adjusting Modules from Other Modules................................................................................................................................................39
Dynamically Changing a Module’s Render Function............................................................................................................................39
Compatibility between Blackfin and SHARC Modules.........................................................................................................................39
Reference Section............................................................................................................................................................................................41
AudioProcessing.h Structures...................................................................................................................................................................41
Module Memory Sections.........................................................................................................................................................................44
Summary of Naming Conventions...........................................................................................................................................................45
Inspector Control Types............................................................................................................................................................................47
XML Format................................................................................................................................................................................................50
Index.................................................................................................................................................................................................................51
3 of 51
Preface
PURPOSE OF THIS MANUAL
The VisualAudio Designer Users’ Guide explains how to use VisualAudio to develop audio processing software for a wide variety of products. The guide describes the graphical interface, provides step-by-step procedures for completing tasks, and contains detailed technical information on how to integrate the generated code into your final product.
Intended Audience
The primary audience for this manual is a programmer who is familiar with Analog Devices, Inc. processors. This manual assumes that the audience can use the VisualDSP++ development environment to develop, build, and debug Digital Signal Processing (DSP) applications for the SHARC or Blackfin processor. 4 of 51
Custom Audio Modules
This document explains how to write an audio processing module for VisualAudio for SHARC processors in the 26x and 36x families, as well as for Blackfin processors in the 53x and 56x families.
Audio modules allow audio processing (sometimes called “post-processing”) to be implemented by making use of a number of smaller, self-contained processing blocks.
The topics are organized as follows.
• “Overview”
• “Numerics on SHARC and Blackfin”
• “Example 1A – Mono parameter scaling”
• “Example 1B – Render function in ASM”
• “Scratch Buffers”
• “Auxiliary Memory for Module Instances”
• “Pointer Aliasing Rules”
• “Meta-variables and Expressions”
• “Modifying Module Parameters”
• “Expression Language Details”
• “Modules with Data of Varying Size”
• “Modules with Variable Numbers of Pins”
• “Other Features of the XML File”
• Custom Bypass Functions”
• “SHARC SIMD Considerations”
• “Adjusting Modules from Other Modules”
• “Dynamically Changing a Module’s Render Function”
• “Compatibility between Blackfin and SHARC Modules”
OVERVIEW
This section includes a brief philosophical review of what motivated certain design decisions, a discussion about the quasi-object orientation inherent in the module concept, a description of usage scenarios and a high-level description of the parts of a module.
Design Philosophy
The module format was designed with the following goals in mind.
• Minimal run-time processor footprint
• CPU efficiency
• Straightforward to write and use
Several key features help accomplish these goals.
• VisualAudio does as much work as possible at compile and assembly time to enable the production DSP code to be lean, while still providing a flexible environment for creating and deploying modules.
• Modules process a block of samples at a time to ensure that the cost of loading and storing state and parameters is incurred only once per block instead of once per sample.
• VisualAudio supports interleaved stereo connections between modules to enable a common use of Single-Instruction, Multiple-Data (SIMD) on the SHARC DSP. This signal type is also supported on the Blackfin, primarily for compatibility with system designs originating on SHARCs.
5 of 51
• VisualAudio supports signals at both the audio sampling rate and a lower “control rate.” This allows slowly-changing control signals to use less memory and MIPS.
• VisualAudio supports a variety of frequency domain signal types, as well as a user-settable FFT size and hop factor for “overlap-add” and “overlap-save” style processing.
• Some of the spirit of object-oriented programming is borrowed, while a lean approach is maintained. Note that C++ is not used.
• To keep the CPU usage (MIPS) of a module relatively constant, a module instance should perform roughly the same operations every time it runs. Assume the module’s worst case CPU usage. The exception is when there are clear modes. In this case, the user can plan in advance the combination of module modes that will be in use at a particular time.
• In keeping with the goals of near-constant CPU usage and minimal memory usage, parameter calculation (such as filter design) is normally pushed forward to design time, and implemented outside the DSP runtime (for example within VisualAudio Designer). Therefore, modules usually do not contain design or initialization code on the DSP. Instead, module instances are normally initialized and designed via static initialization of their state structures (in code generated by VisualAudio Designer or by the user).1
Module Terminology
Each type of processing module is represented by its own module class. These are instantiable; multiple instances of each class may exist at the same time. We use the term module when the distinction between the class and the instance is clear from context. Examples of modules include “Scaler N Smoothed” and “Delay).”
The behavior of modules is adjusted via render variables. These are variables that exist on the DSP as part of the module instance structure. In addition, VisualAudio Designer presents high-level interface variables for each module. Interface variables are those exposed via module inspectors within VisualAudio Designer. An interface variable may correspond directly to a render variable. Alternatively, an interface variable may be mapped to a render variable through some function; for example, translating a delay time in milliseconds to a sample delay. Other possibilities include more complicated dependencies, where one or more interface variables touch one or more render variables.
Render variables are defined in associated .h files detailing the instance structure of each module; interface variables are defined in associated .xml files. Interface variables are sometimes referred to as high-level variables, while render variables are sometimes referred to as low-level variables.
There are three kinds of render variables, differing in restrictions on when they are set:
• Constants are typically set only at design time (i.e. their value doesn’t usually change at run time.)
• Parameters are typically set at design or tuning time from VisualAudio Designer, or by DSP control code
• States can be set by the module’s render function itself, as well as by VisualAudio Designer in tuning mode or by DSP control code.
Within VisualAudio Designer, these restrictions are enforced. On the DSP itself, it is up to the user to abide by these guidelines as appropriate.
The term render variable is used to distinguish it from a meta-variable, which exists only in VisualAudio Designer’s representation of the module, not on the DSP. Thus, the set of interface variables contains some render variables and some meta-variables.
Modules are interconnected via pins. Pins may be designated as either input or output. Either may be of type stereo_pcm, mono_pcm or control. The stereo_pcm and mono_pcm pins are collectively referred to as “audio rate pins,” or simply “audio
1 In stand-alone usage (without VisualAudio Designer) or when modules are implemented in terms of other modules, allocation can be either dynamic or static and initialization DSP code is often included.
6 of 51
pins.” Control rate pins are referred to as “control pins” and are of type control. Frequency domain pins may be of the following types: spectrum_real, spectrum_complex, spectrum_half_real and spectrum_half_complex. These are explained in more detail later.
There are two kinds of modules: those that have a fixed number of pins, and those in which the number of input and/or output pins varies from instance to instance.
A module class may have outputs, but no inputs, in which case it can be thought of as a signal generator (such as a sine wave generator). Or, it can have inputs, but no outputs, and report its results in a state variable (such as a VU meter).
Finally, a module can have neither outputs nor inputs, and can do its work entirely in terms of side effects to itself (modifying its own state) or to other modules (modifying the render variables of other modules). Such a module could be used, for example, in testing other modules, when strictly-repeatable sample-synchronous updates are needed.
Render functions must never write to their inputs. To see why this is true, consider a module whose output fans out to several other modules. If the first module wrote to its input, it would corrupt the input to the second module. However, the VisualAudio Designer routing algorithm knows the overall connection between audio modules and may reuse the same patch buffer for the input and output of a module, when it is safe. For more details, see Pointer Aliasing Rules below.
Module Usage Scenarios
There are two ways that VisualAudio modules can be used:
• In a drag-and-drop fashion from VisualAudio Designer - Memory allocation, parameter setting and calling of the render function are handled automatically.
• As C-callable functions in a stand-alone library - Memory allocation, parameter setting and calling of the render function are all handled by the user’s C or assembly code.
Even if a module is used in drag-and-drop fashion, its render variables may be modified in the DSP program’s control code (sometimes referred to as “user control code.”) Similarly, a module used in a drag-and-drop fashion may include, in its implementation, a render function that calls other render functions using the stand-alone style.
This document contains information on developing modules that may be used in either style of usage. For more information on usage, see the document VisualAudio Module Library Usage Guide. For more information on the particular modules supplied by VisualAudio, see VisualAudio Module Library Reference for Blackfin and VisualAudio Module Library Reference for SHARC.
Module Modes
When used within a layout generated by VisualAudio Designer1, a module may be in one of four modes. These can be set at runtime with the following function:
AMFSetModuleStatus(AMF_Module *module, AMF_ModuleStatus status)
The possible status values and their meanings are given below.
• AMFModuleStatus_ACTIVE. The module processes its inputs and writes its outputs via its render function each time it is run. This is the default mode. Note that a module may have several alternative render functions, but one must be specified as the default.
• AMFModuleStatus_INACTIVE. The module is not run. This implies that its outputs are not written, leaving their contents undefined.
• AMFModuleStatus_MUTED. The module's outputs are zeroed each time it is run. This behavior is provided automatically. You need not write any code to implement this mode.
1 More specifically, when used with the VisualAudio Layout Support library.
7 of 51
• AMFModuleStatus_BYPASSED. The module performs the bypass function, which means that its input(s) are copied to its output(s) each time it is run. The default algorithm copies audio inputs to audio outputs, copies signal inputs to signal outputs, and mutes unused outputs. Where there is a mono/stereo mismatch, stereo is converted to mono by adding the channels and dividing by two; mono is converted to stereo by duplicating the channel. Alternatively, the module designer may provide a custom bypass function. For more information, see How to Write a Custom Bypass Function below.
The default bypass algorithm copies the Nth input pin of a given type to the Nth output pin of the same type. For example, the 3rd control pin input is copied to the 3rd control pin output. If there are more output pins than input pins, the remainder are muted. Note that for the purposes of bypass, stereo and mono pins are considered the same type. If a mono input matches a stereo output, the mono input is duplicated on both channels. If a stereo input matches a mono output, the stereo channels are added and divided by 2.
Parts of a Module
A module consists of these parts:
• A header (.h) file that defines the run-time interface to the module, including the instance structure typedef. The name of this file must be the same as the module name with .h (for example, AMF_Scaler.h).
• The module’s run-time DSP code, in source or binary form (e.g., to protect any intellectual property). The VisualAudio Module Library is delivered in binary form as a VisualDSP++ .dlb file, and the source is also included. If delivered in source form, the module must contain the following two parts:
• The module’s render function, which implements the module’s primary function
• The module’s class object, which describes the module to the run-time system
• A .xml file that describes the module to VisualAudio Designer in detail. This file is not required if the module is never used with VisualAudio Designer. The name of this file must be the same as the module name, with .xml appended (for example, AMF_Scaler.xml where “AMF” stands for Audio Module Format). The .xml file includes information about what files constitute the module’s run-time and header files, as well as information about the module’s parameters, and may also include simple design formulas.
How to Add a Module to VisualAudio Designer
To make a custom SHARC module available to VisualAudio Designer, create a directory (we’ll call it xxx) and put the XML, include, source files and object files1 in sub-directories. For the SHARC, the subdirectories should be:
• XML files in xxx\SHARC\XML\
• Header files in xxx\SHARC\Include\
• Source files in xxx\SHARC\Source\
• Object files in xxx\SHARC\Lib
For the Blackfin, they should be:
• XML files in xxx\Blackfin\XML\
• Header files in xxx\Blackfin\Include\
• Source files in xxx\Blackfin\Source\
• Object files in xxx\Blackfin\Lib
Where xxx is your Modules directory. You must then add your Modules directory to the list of directories searched by VisualAudio Designer. See the VisualAudio Designer User's Guide for details.
1 Third parties can protect their IP by delivering it as a library (a .dlb). Alternatively, they can deliver it is as a pre-compiled or pre-assembled object file (a .doj).
8 of 51
You must add your custom module source files to the VisualDSP++ project (.dpj) file for your platform. In contrast, when a module is included in object form (.dlb or .doj), it is automatically added to the linker list via the VALinkerCmds.txt file.
NUMERICS ON THE BLACKFIN AND SHARC
The primary difference between Blackfin and SHARC modules is the use of floating point on the SHARC. On the Blackfin, floating point is not available in hardware; hence Blackfin modules typically operate in fixed point. The basic VisualAudio signal type on the Blackfin is fract32, a 32-bit 1.31 format fraction. The basic VisualAudio signal type on the SHARC is a float, a 32-bit floating point number. To ease the task of moving between SHARC and Blackfin, VisualAudio defines a type AMF_Signal, which is fract32 for Blackfin and float for SHARC.
Most SHARC modules use floating point internally. However, extended precision SHARC modules may use fixed point internally. Most Blackfin modules use fixed point internally.
A number of conventions have been established for fixed-point processing on the Blackfin. We recommend that custom modules obey these conventions for maximum compatibility:
The default format for fixed point coefficients is 1.31. Coefficients which perform a “volume scaling” can be 16 bits (typically 1.15 format), so that faster 16x32 multiplication can be used (as opposed to 32x32), since a volume-like scale tends not to need to be represented with an extremely high precision. Smoothing of 16-bit coefficients may need to be performed at 32 bits (to allow the smoothing to move at very slow smoothing rates), but the top 16 bits can still be used for doing the volume scaling cheaply.
Headroom in signals is assumed to be managed by the layout creator, not by the module or by VisualAudio. Therefore, except where noted, a Blackfin module assumes 1.31 input and output signals, and for compatibility a SHARC module assumes signals where 1.0f corresponds to maximum amplitude (though clipping to +/- 1.0 is only implemented at the output). Saturating arithmetic is used in fixed point modules.
In fixed point modules, multiplications implemented to “31-bit” precision (i.e. discarding the low order product as a speed optimization) may be used as a satisfactory substitute for full 32x32 multiplications.
16 bit types (fract16 and int16 ) as module variables are not supported on the SHARC in VisualAudio.
The module implementer is responsible for creating correct alignment in the module state structure, if necessary (via padding and/or ordering). This is an issue only with Blackfin modules. The structures allocated by VisualAudio Designer can be assumed to be aligned to 32-bit boundaries.
EXAMPLE 1A – MONO PARAMETRIC SCALING
The following example shows a parametric scaling of a mono signal, for both SHARC and Blackfin versions of VisualAudio
Example 1A Header File: AMF_Scaler.h
The example module’s header file is shown below, for the SHARC or Blackfin version of VisualAudio:
/***** Begin AMF_Scaler.h *******/
// Include header file with base class definitions:
#include "AudioProcessing.h"
// Instance structure typedef
9 of 51
typedef struct {
AMF_Module b;
// Parameters
AMF_Signal amplitude;
} AMF_Scaler;
// Class object declaration
extern const AMF_ModuleClass AMFClassScaler;
/**** End AMF_Scaler.h *****/
Notice that the instance structure begins with an embedded struct of type AMF_Module. All module instance structures must begin in this manner (this allows any module’s struct to be interpreted as an AMF_Module, hence implementing a form of inheritance). This struct is followed by a single render variable, amplitude.
The structure for the Blackfin and SHARC versions of the module are identical, except for the definition of AMF_Signal as fract32 instead of float in AudioProcessing.h.
Example 1A Code File: AMF_Scaler.c
The example module’s C code file is AMF_Scaler.c. The first half of the C file for the SHARC version of the module is listed below and analyzed in detail, with comparisons to the Blackfin version as necessary.
/****** Begin AMF_Scaler.c *********/
#include "AMF_Scaler.h" // The module's header file
#pragma optimize_for_speed // VisualDSP++ directive
SEG_MOD_FAST_CODE void
AMF_Scaler_Render(
AMF_Scaler *restrict instance,
AMF_Signal * restrict * buffers,
int tickSize)
{
int i;
AMF_Signal *in = buffers[0];
AMF_Signal *out = buffers[1];
AMF_Signal amplitude = instance->amplitude;
#pragma SIMD_for
for (i=0; iamplitude;
#pragma SIMD_for
for (i=0; iamplitude;
for (i=0; i tag with value 2.
To make it easy to supply values for the type vector, the following macros are supplied:
#define AMF_StereoPin(whichPin) \
(AMFPinType_STEREO<<(whichPin*4))
#define AMF_ControlPin(whichPin) \
(AMFPinType_CONTROL<<(whichPin*4))
#define AMF_MonoPin(whichPin) (0)
#define AMF_SpectrumRealPin(whichPin) \
(AMFPinType_SPECTRUM_REAL<<(whichPin*4))
#define AMF_SpectrumComplexPin(whichPin) \
(AMFPinType_SPECTRUM_COMPLEX<<(whichPin*4))
#define AMF_SpectrumHalfRealPin(whichPin) \
(AMFPinType_SPECTRUM_HALF_REAL<<(whichPin*4))
#define AMF_SpectrumHalfComplexPin(whichPin) \
(AMFPinType_SPECTRUM_HALF_COMPLEX<<(whichPin*4))
Type descriptors can then be assembled by bitwise OR’ing of these macros. Note that the whichPin argument is zero-based. For example, if a module has one mono input followed by one stereo input, its input type designator could be written as:
(AMF_MonoPin(0) | AMF_StereoPin(1))
Alternatively, it could be written directly as 0x10.
If there are more than eight pins, then the high order nibble is assumed to be sticky and applies to all pins beyond eight. However, there are situations where this convention is inadequate, such as when a pin greater than the 8th has a type differing from the 8th. For these situations, an indirect form is available as follows:
13 of 51
If the AMF_ModuleClass flags field includes the bit AMFModuleClassFlag_INDIRECT_INPUT_PIN_TYPE, then the input type descriptor is actually a pointer to an array of sufficient length to support bit vectors for all input pins. Similarly, if the flags include the bit AMFModuleClassFlag_INDIRECT_OUTPUT_PIN_TYPE, then the output type descriptor is actually a pointer to an array of sufficient length to support bit vectors for all input pins.
In modules with variable number of pins (described in a later section of this document), the input and output type descriptors are in the instance, rather than the class.
Example 1A XML File: AMF_Scaler.xml
The .xml file describes the module to VisualAudio Designer. In this discussion, we assume a minimal familiarity with XML. Please note that all module xml element type attributes (i.e. type = “string”, type = “float” etc.) are optional as of VisualAudio 1.6 and therefore, are not shown in the examples below.
When creating a custom module, we recommend copying the XML file from an existing module, renaming the XML file, and modifying it.
At the outermost level, the XML file looks like this:
. . .
It begins by telling the XML parser where to find the VisualAudio Designer schema, which is used to validate the file.1 Validating the file ensures that it has all the information needed by VisualAudio Designer, that it is structured correctly, that the fields are listed in the proper order, and that it contains legal values for the required fields. The actual module definition is inside the body of the tag, which includes the information detailed below.
Module Fields
A module has several different self-description tags
• The